The primordial elements are four in number: air, fire, earth, and water; and that it is from their coherence to one another under the molding power of nature that the qualities of things are produced according to different classes. And, in fact, we see only that all which comes to birth is produced by them, but also that nothing can be nourished without their influence, nor grow, nor be preserved.
—Vitruvius1
Changing Primordial Elements in the Twenty-First Century
Ever since the harnessing of fire, humans have nurtured an independence from the sun that has been wielded as a foundational force in shaping architecture. Fire has been grounded in architecture through its historic symbolic value and enduring contemporary use, from its warming of the domestic hearth to its voracious appetite for oxidizing fuels.2 Relative to the three other primordial elements—air, water, and earth—the capacity to use fire as an ally has vastly extended human power over materials and landscapes. Fire fights against material resistance, either rapidly accelerating or delaying the return of materials to the other three elements through complete or partial acts of combustion. On agricultural fields, fire has long-since aided the return of crop residues to air and earth, while in pyrolysis chambers, fire is used to combust biomass, rubber, and plastic materials into carbon-rich solids and gases.3
Fire is also a powerful partner in strengthening organic and inorganic materials, paving the way to lodge impurities, binders, and admixtures into homogenous material matrices and generating recombinant materials. Fire is wielded as a disciplinary agent in metal tempering, in which fire toughens metals by heating them to temperatures just below their melting points before cooling. Applied in collaboration with controlled pressure, fire is a reliable accomplice in thermally compressing weakened or aging materials into mechanically superior products. Today, to compensate for the design of short-sighted material lives and large volumes of solid waste, fire has become essential in fusing and re-fusing everything from single-use plastics to discarded textiles to agricultural biomass residues in order to generate longer-life products. However, given fire’s varying capacity to substantially exacerbate or remediate environmental toxicity (alongside circular material practices), broader ecological frameworks are urgently needed to recalibrate cultures of fire around material production, transformation, and degradation.
Material transformation and circularity in ancient human cultures are often embedded in cosmological belief systems that are both constituted and driven by air, water, earth, and fire. Clay pottery in the ancient Mayan worldview, for instance, represented the metabolic interaction of clay, from the aquatic underworld, with the upperworld element of fire.4 In the Aboriginal Australian cosmic concept of “country,” material components of animate bodies like flora and fauna are inseparable from the inanimate components of the ecology through which fire, in concert with the other elements, facilitated exchange.5 And in the western tradition, both the human body and building materials are underpinned by these elemental relationships, and are not seen to be dissimilar from the material vitality of soil.6
According to Vitruvius: “Those [materials] which contain a larger proportion of air, are soft; of water, are tough from the moisture; of earth, hard; and of fire, more brittle.”7 Accordingly, “earthy” juices like blood, milk, sweat, urine, and tears course through the human body. Continuously heat-tempered around 37℃ by its internal core organs and muscles, healthy human blood contains known compositional ratios of water, oxygen, and solid “earthy” components like red and white blood cells and platelets. Just as human health is directly impacted by the maintenance of primordial ratios, the health of soil is dependent on a continuous exchange and storage of fluids: “earthy” materials dissolved in water form a spectrum of juices that flow and saturate the internal air cavities within soil.
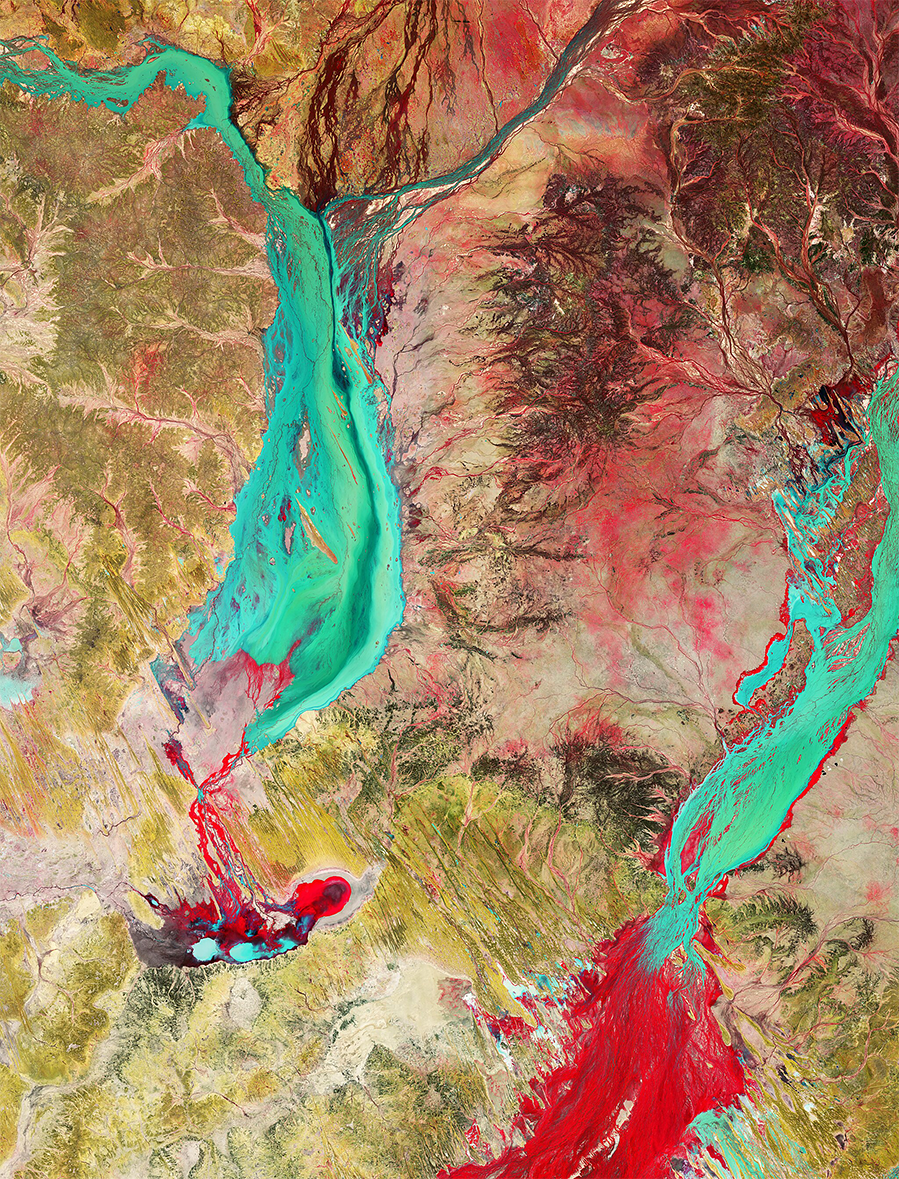
Channel Country in Queensland, Australia showing vegetation (bright red), mud and sediments in the Georgina and Diamantina Rivers (cyan), and desert, bare soil, and rocks (green). Source: European Space Agency.
Two millennia after Vitruvius’s treatise, the qualitative shift brought about by the climatic and geological impacts associated with the Anthropocene in the very nature of these four elements offers new predicaments. What constitutes the identity and health of twenty-first century materials? What should the role of humans be in the responsible management and remediation of material ecologies? Not only has the fear of fire’s strength and spread in built environments fueled low biodiversity in building materials, but it has also, with the use of flame retardants, produced highly polluted indoor air.8
Furthermore, since the first commercial production of synthetic plastic in 1907, 200 million metric tons of plastic waste have entered our oceans, with an estimated eleven million metric tons added every year.9 With 70% of our planet’s surface covered in oceanic water, which has the capacity to break down, mix, and transport materials across long distances, the significant quantities of plastics that have entered the ocean are now distributed widely across the planet. This vast range of small particles, less than five millimeters in diameter, are gradually reconstituting everything from the chemical composition of sand to atmospheric quality and the human gut microbiome.10
If plastics dominate the surface and depths of our oceans, 80% of ocean waste offloading has reconstituted the bottom of rivers and canals with toxic industrial waste accumulation.11 The impact of changing ocean floor topography and chemistry continues to be associated with coastal erosion, bioaccumulative pollution, and water compositional changes that underpin the health of marine ecosystems and coastal habitats.12 This has resulted in the rising frequency of new primordial phenomena, including “dead zones” with reduced oxygen levels or highly toxic “red tide” algal blooms.13 As synthetic and chemical components infiltrate interconnected material cycles, there are increasingly limited opportunities to escape such new natures.
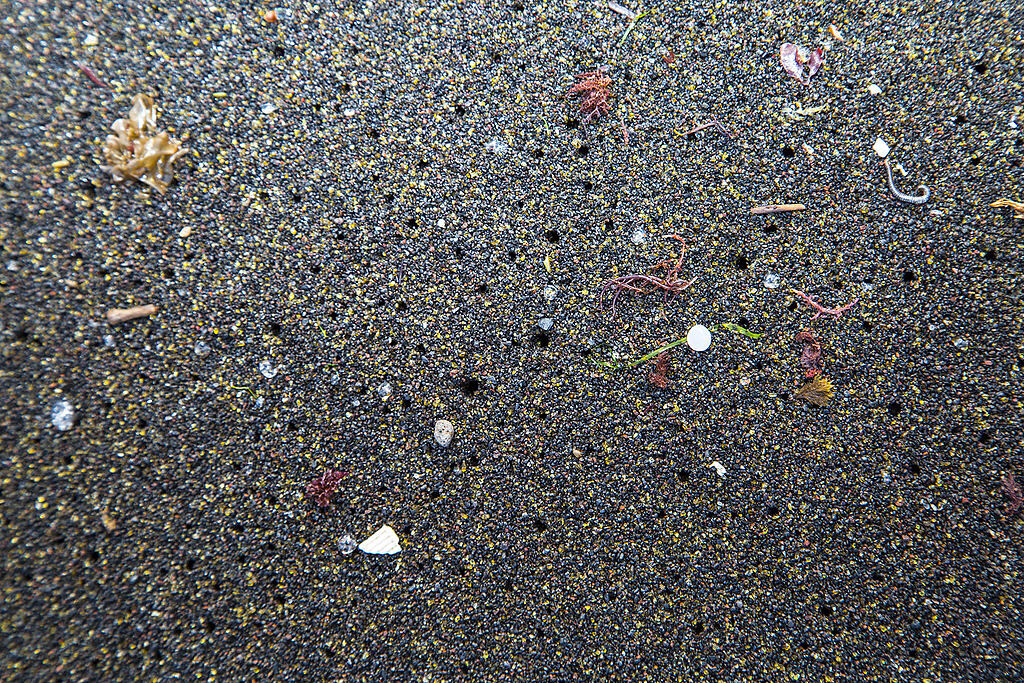
Microplastics appear almost indistinguishable from soil minerals in the Azores. Source: Peter Charaf, Creative Commons.
Given the reality of such material ecologies, today’s existing hierarchy of design strategies for material transformation—cascading from reuse to recycling, landfill, and incineration—require new paradigms of design, entrepreneurship, and economic investment. Akin to recycling, coursing through the veins of the material reuse paradigm is a paradoxical goal of designing materials to last forever, which typically requires the use of energy-intensive, rare, or toxic processes that often compromise healthy, trophic activity within material ecosystems. Material design propositions that do not engage the reality of the surrounding material muck are, at best, short-sighted, and, at worst, a privileged side-step.
It is in this spirit of “muck,” which includes material surplus, contamination, microparticles, and leachates, that fire exists as a force to redress material displacement across twenty-first-century ecologies. Whereas the use of fire as a universal solvent has underpinned the successful control and scaling of inorganic material production, it has had disastrous impacts when transposed onto forestry and agricultural landscapes, diverse material families, and climates, leading to rising levels of combustion by-products as well as ecological pollution. To address this, the careful spatialization, timing, and intensity of fire regimes within an ecological framework offers opportunities for anticipating fire’s spread and engaging its capacity to drive material regeneration. Similar to the art of metal tempering, the strategic use of fire in the production of fused and re-fused materials is critical for ensuring that such processes do not merely compound negative life-cycle impacts in order to achieve short-term performance gains.
Fire as a Universal Solvent
From a material processing perspective, Vitruvius’s description of fire bears close kinship to today’s contemporary use of fire as a levelling force in material processing. For Vitruvius, fire is represented as a “universal solvent” capable of dissolving the natural resistance of a material with its fiery force, weakening the material to a state of surrender. Under firing processes, chemical bonds of varying strengths are broken and rapidly forced to react with oxygen. In his examination of lime production, for instance, Vitruvius demonstrates that limestone’s capacity to bond with sand can only be achieved after it has undergone heating until exhaustion. This process of relentless heating lays bare limestone’s pores for oxidation processes, rendering it ready to react with water to form a new material.14 In the burning of coal and inorganic minerals like limestone, oxidation occurs at temperatures of between 1300–1500℃, while for organic biomass this occurs between 200–350℃.
Paradoxically, social perceptions of safety and control appear to improve alongside higher temperatures. Fear of fire within human habitats is largely dominated by lower band temperatures around 200℃, at which point the ignition of fibrous and thatch materials occurs and fire spreads quickly in open-air. As temperatures rise to 500–700℃, which is around when common wood biomass like pine or oak burn, human anxiety around fire dissipates and becomes more closely associated with thermal and psychological comfort. At different scales and in different cultures, humans gathering around the domestic hearth and communal bonfires complement domestic rituals of cooking, marriage, socialization, rest, and death.15
At the next higher temperature band of 800–1100℃, fire has been creatively wielded as a tool for mineral and metal fusion. Not only does the traditional firing of earth bricks rely on the fusion of lime and sand at these temperatures, but that firing has been used to overcome the scarcity of certain material properties. For example, given the rarity of the color blue in nature, ancient Egyptians and Romans developed a process that leveraged fire to heat sand, the flower of natron, and copper to temperatures of 800–900℃ in a ceramic vessel. At such temperatures, all three inorganic materials are forced “to mix in each other’s sweat and relinquish their peculiar qualities,” yielding rarefied, permanent blue pigments.16
On a material scale, social perceptions of fire in relation to human safety have directly driven material design and integration logics. Enforced by performance standards and fire safety building codes, toxic chemical additives are integrated into the material matrix or surfaces of almost all materials today to prevent and inhibit the spread of fire. Despite growing awareness of their persistent toxicity, the continued growth of the $7.6 billion global market for fire retardants reflects a deeply seated fear of material vulnerability to heat and weathering.17 In contrast to the increasing volume of materials, often laden with toxic chemicals, that eventually make their way to landfills or oceans, fire can be a counter force in curbing material pollution. Here, the thermal refusion of mixed material streams to generate higher density products shifts the pattern and scale of pollutant pathways, prolonging the participation of such materials in the market economy. However, given that the interaction of “fused” material products with primordial elements can negatively impact indoor and outdoor environmental quality, new spatialization paradigms, integration design, and servicing models for such composite material systems are key to ensuring non-toxic decomposition and the ongoing maintenance of the built fabric.
Fire Farming
In contrast to the use of fire as a ravenous force for heat-tempering and refusing materials, writer Bruce Pascoe describes the Aboriginal conceptualization of fire as a regenerative agent.18 Similar to the low-grade fire burning regimes practiced by Native Americans, Aboriginal Australians developed practices of “cool burning” to drive the abundance of domesticated flora and fauna. Through the quick and partial burning of relatively small amounts of strategically spaced biomass and underbrush at specific times, coupled with an understanding of prevailing wind and dew patterns that helped control direction and spread, the risk of wildfire was eliminated. This knowledge around the timing of fires was heavily informed by an intimate familiarity with soils, memory of the last cool burn within the context of plant life cycles, and the observation of weather patterns. Fire planning regimes were also critically preceded by sharing schedules and knowledge among neighbors.19
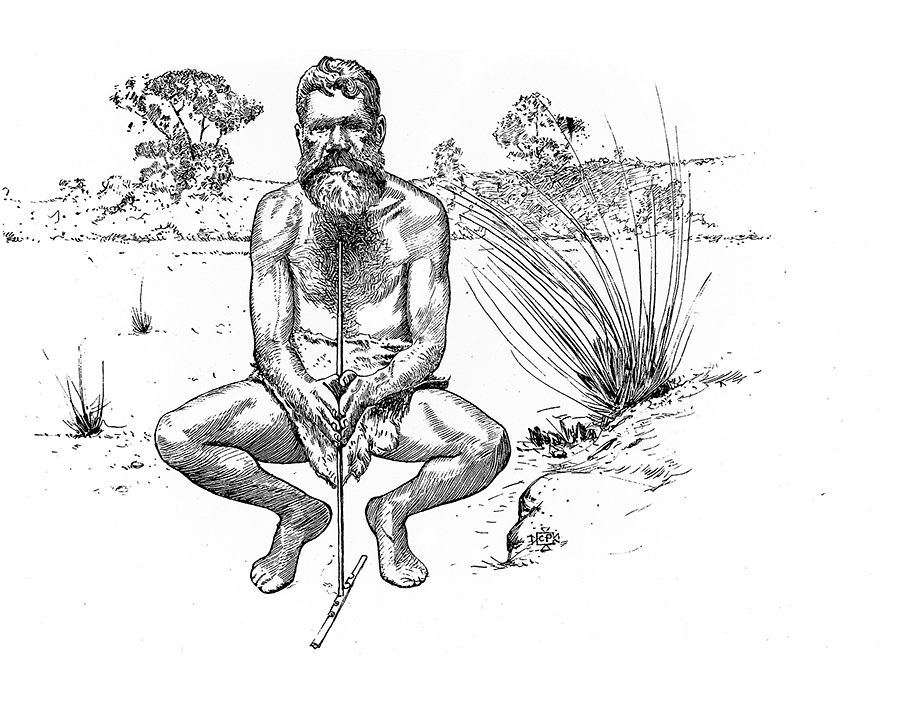
Fire-making by an Aboriginal man in New South Wales. Source: Wellcome Collection, Creative Commons.
Across country, knowledge of the present fertility of soil also informed where cool-burning was done. Cool burns were performed on fertile, productive soils, while unproductive soils were left to remain as forestlands. Within agriculturally productive patches of land, the regenerative impacts of cool burning both forced seeds to crack open and activated preserved biomass roots and moisture below ground. Cool fires gave such lands a “kiss of life.” Here, the spurt of shorter, nutritious, “sweet feed” attracted fauna like cattle and kangaroos for hunting.20
In the context of today’s catastrophic wildfires, new platforms and avenues are emerging to overcome barriers to cool fire regimes—barriers including farm fences, land use restrictions, property boundaries, as well as the complex politics of land ownership and air quality.21 Beyond fire management, the knowledge of plant cycles fundamental to these burning practices is part of deeply interwoven agricultural and architectural traditions intrinsic to vernacular building practices. Literacy around soil and plant life cycles plays a critical role in determining how biogenic material harvesting can be designed and timed to promote the health and resiliency of soil ecosystems.22 Such approaches extend the capacity of material designers and specifiers to stimulate a demand side that supports species abundance and enhances biodiversity.
Flameless Fire
Whereas fire relies on the burning of a fuel in the presence of oxygen, heat relies on the transfer of thermal energy between material media. On a global scale, differences in the heat content of primordial elements drive local and planetary climatic and geologic patterns that shape microclimatic flows across built habitats. At the building scale, heat exchange between primordial elements in the building’s material systems determines the thermal conditions of indoor spaces and their surrounding outdoor ecologies. Across diverse climates, long-standing building practices have used these primordial elements—water, earth, air—in concert with mineral and organic materials to serve as thermal storage within building walls and roofs in order to absorb, slow down, and control the release of heat.23
In terms of material production, relatively low-grade heat (just under 180℃), in combination with pressure, helps create a broad category of materials made from reconstituted wood, agricultural or forestry residues, plastics, textiles, and diverse aggregates. Typically preceded by shredding, milling, and pulverization, such approaches require a mechanical reduction of material into constituent parts and a partial destruction of structural bonds. A higher degree of erasure of the material’s former identity and the capacity to improve standardized “bits,” including small chips, particles, fiber lengths, pellets, and fluff, often corresponds positively with the quality of the new material and its near-term market demand. In addition to the labor and energy requirements for mechanical degradation, material “bits” then require glues to piece them back together. Due to the negative impacts of synthetic glue concentrates in factory settings and the persistent off-gassing of harmful chemicals from large surface areas of thermally pressed materials, the toxicity and chemical behavior of glues under heat is critical to consider in the design of healthy indoor environments.
Since the late nineteenth century, glues used in wood and reconstituted wood have been dominated by toxic petroleum-based urea-formaldehyde, isocyanate, and other formaldehyde-based adhesive binders that are responsible for off-gassing volatile organic chemicals (VOCs) into indoor environments.24 Furthermore, across a growing myriad of material recombination substrates—wood-biomass, mixed plastic, wood-plastic, plastic-mineral aggregate, or bioplastics—increasing percentages of glue can be used to compensate for substrate variability, non-standardization, and mechanical strength. From a circularity perspective, the consequent impregnation of toxic glues into pressed material products merely delays and multiplies problems of toxicity and non-biodegradability. The use of synthetic glues in such materials often results in toxic, higher thermal pressing conditions and disposal practices that drive up the embodied carbon emissions associated with the manufacturing, maintenance, and degradation of materials. In rethinking the tradeoffs between short-term material performance gains and long-term ecological toxicity, new criteria around end-of-life processes should be centered in material design practices.
The use of natural binders as an alternative to synthetic glues offers a promising approach to curbing high material carbon footprints and long-term toxicity. Termed “binderless boards,” the thermal pressing of biomass residues leverages a relatively gentle intensity of heat to force the internal bonding of naturally abundant and non-toxic glues. In plant cell walls, a complex conglomeration of polymers, known as lignin molecules, wrap around cellulose sugar molecules like packing peanuts. Responsible for reinforcing, binding, and connecting plant walls, lignin plays a critical role in strengthening plant architectures. Found in abundance in skins, husks, peels, stems, and barks, lignin is part of a plant’s defense system to protect cells from harmful molecules, invasive organisms, and climatic conditions. Compared to other plant protective barriers, which are typically composed of less than 30% lignin, the husk of mature coconut contains over 40% lignin.25
When thermal pressing a wide range of plant skins and materials, lignin binders soften and dry out as temperatures approach 140℃ and a chemical cross-linking occurs.26 This irreversible condensation of lignin bonds formed under low-grade heat replaces the need for synthetic glues in plant-based thermally-pressed composites.27 In contrast to synthetic glues, if left uncoated, pressed biocomposite materials remain “breathable,” maintaining unique porous matrices that improve their capacity to hold moisture and cool via evaporation.28
Biological Fires
Relative to material transformations stemming from heat and firing, biological digestion offers another frontier for material recycling. In collaboration with other species, fungi have historically served as the ubiquitous agent both for decomposing dead matter and for enabling living matter to embark on new conquests in uncharted material environments.29 In agriculture, fungal activity enhances all forms of plant activity, including photosynthetic systems, the maintenance of soil structure, and the rapid decomposition of dead plant matter through enzymatic activity.30 Mycelium—the vegetative part of fungi—has the capacity to digest a vast variety of materials, including plastics, and is an effective biomass-to-biocomposite material technology. Feeding on tightly bound lignin and cellulose components of organic matter, mycelium can metabolize a diverse array of materials, ranging from hydrocarbons to carbohydrate chains, to generate resilient chitin-bound green building materials at room temperatures of 18–24℃. Effectively “growing” materials under low-energy conditions, these chitin molecules can be used in non-toxic bioproducts.
Like fungi, fire and heat may be important allies in directly sequestering, transforming, and maintaining twenty-first century material muck. As one of nature’s best chemists, fire both deconstructs and renews our material worlds, while heat continuously shapes and conditions it. Stemming from fire’s pervasive capacity to force reactions between material constituents, fire has become an important tool to engage when prescribing and spatializing material systems within the built environment. Unlike contemporary energy and material paradigms that rely disproportionately on active mechanical heating and sealed environments, linking the design of healthy materials with thermodynamic regimes is central to accelerating non-toxic circular material practices.
M. H. Morgan and H. L. Warren, Vitruvius: the ten books on architecture (Cambridge, MA: Harvard University Press, 1914), 225.
Luis Fernández-Galiano, Fire and memory: On Architecture and Energy (Cambridge, MA: MIT Press, 2000).
C. J. Wrasman et al., “Catalytic pyrolysis as a platform technology for supporting the circular carbon economy,” Nature Catalysis 6, no. 7 (2023): 563-573.
Patrice Bonnafoux, “Waters, droughts, and early Classic Maya worldviews,” Ecology, power, and religion in Maya landscapes, Acta Mesoamericana 23 (2011): 31-48.
Bruce Pascoe and B. Gammage, Country: Future Fire, Future Farming (Thames & Hudson Australia, 2021), 1.
Pascoe and Gammage, Country, 241.
Pascoe and Gammage, 46.
E. Uhde and T. Salthammer, “Impact of reaction products from building materials and furnishings on indoor air quality—a review of recent advances in indoor chemistry,” Atmospheric Environment 41, no. 15 (2007): 3111-3128.
J. L. Meikle, American plastic: A Cultural History (Rutgers University Press, 1995), 40; K. Pabortsava and R. S. Lampitt, “High concentrations of plastic hidden beneath the surface of the Atlantic Ocean,” Nature Communications 11, no. 1 (2020), 4073.
G. Fackelmann and S. Sommer, “Microplastics and the gut microbiome: how chronically exposed species may suffer from gut dysbiosis,” Marine Pollution Bulletin 143 (2019): 193-203.
M. G. Gross, “Effects of waste disposal operations in estuaries and the coastal ocean,” Annual Review of Earth and Planetary Sciences 6, no. 1 (1978): 127-143.
Gross, “Effects of waste disposal operations.”
N. J. Bennett et al., “Environmental (in) justice in the Anthropocene ocean,” Marine Policy 147 (2023): 105383.
Morgan and Warren, Vitruvius, 46.
Fernández-Galiano, Fire and memory, 8-11.
Morgan and Warren, Vitruvius, 218-219.
“Global Flame Retardant Market By Type” (October 2023). See ➝.
Pascoe and Gammage, Country.
Bruce Pascoe, Dark emu black seeds: Agriculture or accident? (Magabala Books, 2014), 227-228.
R. Bliege Bird et al., “Aboriginal hunting buffers climate-driven fire-size variability in Australia’s spinifex grasslands,” Proceedings of the National Academy of Sciences 109, no. 26 (2012), 10287-10292.
L. Penniman, Farming while black: Soul fire farm’s practical guide to liberation on the land (Chelsea Green Publishing, 2018); D. W. Crowder et al., “Conserving and promoting evenness: organic farming and fire‐based wildland management as case studies,” Ecology 93, no. 9 (2012), 2001-2007.
P. Osborne et al., “A trait-based approach to both forestry and timber building can synchronise forest harvest and resilience,” PNAS nexus 2, no. 8 (2023).
M. Azarbayjani and D. J. Thaddeus, “High Comfort–Low Impact: Integration of Thermal Mass in Pursuit of Designing Sustainable Buildings,” in Achieving Building Comfort by Natural Means, ed. Ali Sayigh (Cham: Springer International Publishing, 2022), 47-97.
Steve Brown, “Occurrence of Volatile Organic Compounds in Indoor Air,” in Organic Indoor Air Pollutants: Occurrence-Measurement-Evaluation, ed.Tunga Salthammer (Weinheim, Germany: Wiley-VCH Verlag, 1999); Lilli Kirkeskov Jensen et al., “Health Evaluation of Volatile Organic Compound (VOC) Emissions from Wood and Wood-Based Materials,” Archives of Environmental Health 56, no. 5 (Sept–Oct 2001): 419-432.
Jan E. G. Van Dam et al., “Process for production of high density/high performance binderless boards from whole coconut husk: Part 1: Lignin as intrinsic thermosetting binder resin,” Industrial Crops and {roducts 19, no. 3 (2004), 207-216.
Jan E. G. Van Dam, Martien J. A. van den Oever, and Edwin R. P. Keijsers, “Production process for high density high performance binderless boards from whole coconut husk,” Industrial Crops and Products 20, no. 1 (2004), 97-101.
J. Xu et al., “Manufacture and properties of low-density binderless particleboard from kenaf core,” Journal of Wood Science 50 (2004): 62-67.; R. Hashim et al., “Characterization of raw materials and manufactured binderless particleboard from oil palm biomass,” Materials & Design 32, no. 1 (2011): 246-254; Van Dam et al., “Production process for high density high performance binderless boards,” 2004.
Mae-ling Lokko and Alexandra Rempel, “Intrinsic Evaporative Cooling with Natural Ventilation and Shading for Adaptive Thermal Comfort in Tropical Buildings” (International Building Physics Conference, 2018).
Stefan Rensing, “Great moments in evolution: the conquest of land by plants,” Current opinion in plant biology 42 (2018): 50-51; Xiaoliang Fu et al., “Fungal succession during mammalian cadaver decomposition and potential forensic implications,” Nature Scientific Reports 9, no. 1 (2019): 2-5.
Geoffrey M. Gadd, “Mycotransformation of organic and inorganic substrates,” Mycologist 18, no. 2 (2004): 60-70.
Material Acts is a collaboration between e-flux Architecture and Craft Contemporary within the context of the eponymous exhibition, curated by Kate Yeh Chiu and Jia Yi Gu as part of Getty PST ART: Art & Science Collide.