150 years after the start of the industrial revolution, energy consumption per capita has grown almost five-fold in Europe.1 And while the slow paradigm shift to carbon neutrality has started, fossil fuels still affect how we design, build, and inhabit space. The indoor air we breathe is often mechanically moved and tempered by energy-guzzling, vulnerable HVAC systems, all to sustain year-round “optimal” thermal comfort.2 Heating and cooling account for half of the EU’s energy consumption, with 75% of this energy still being sourced from fossil fuels.3 The knee-jerk reaction to this has thus far usually been to tighten regulations on insulation and structural performance. This is what current EU regulations are aiming for.4 But it’s the wrong path.
Within the context of these knee-jerk policies, extra insulation is often added to the exterior of buildings in cold climates to improve their energy efficiency. But this can compromise the structure’s resilience to humidity.5 This insulation has also often proven ineffective in reducing overall energy consumption; there are indications that insulation retrofitting has a rebound effect, where energy efficiency does not affect overall consumption.6 In other words, not only do these retrofits risk causing structural moisture problems, but the anticipated energy savings of a thermally better performing structure are often countered by a heightened expectation of comfort from its user. We dwellers are simply demanding too much from buildings, and not enough from ourselves.
The current expectations of comfort shared by the wealthy Western world, and the degree to which we expect indoor space to offer that comfort, is not necessarily the result of an imperceptibly slow cultural shift.7 Helsinki is exemplary of how rapidly such a shift can take place.
Firewood was the prevalent heat source in Northern European countries through the turn of the twentieth century. Indeed, in Finland, firewood—which is also the country’s main natural resource—would remain the primary energy source until 1960. Starting in the 1900s, however, steam-powered transportation allowed for the import of a new fuel source—coal—and the technology to use it—central boiler technologies—from wealthier parts of continental Europe. Buildings in coastal cities such as Helsinki quickly began to be designed to be heated with coke, a coal-based fuel with a high carbon content and few impurities.8
During the eighteenth and nineteenth centuries, domestic stoves had been designed to effectively exploit the heat of firewood. While passing through a network of ducts on its way out, smoke would slowly heat the stove’s masonry structure, and then slowly release heat into the room. The dimensions of these ducts and the masonry surrounding them were designed to optimize both the airflow and the heat storage-to-release ratio—allowing the stove to store heat and release it at the desired degree.
Keeping warm required maintaining one’s fuel supply, and, when necessary, making a trip to one of the many marketplaces to purchase a horse-cartful of firewood (which was subject to both seasonal and market-driven price volatility). Heating stoves was a daily household chore, which included tasks like carrying wood from the cellar and, once a day, lighting and fuelling the masonry stove in each room that needed heating.9 When lighting the stove, inhabitants became accustomed to paying attention to combustion levels, adjusting the damper and the room’s ventilation accordingly. This act of heating thus also renewed the air in the room. After the fire had gone out, the ventilation would be closed to allow fresh air to be slowly consumed while inhabitants enjoyed the heat stored and radiated by the masonry stove and adjacent walls. As a container of oxygen, rooms thus often had rather high ceilings.
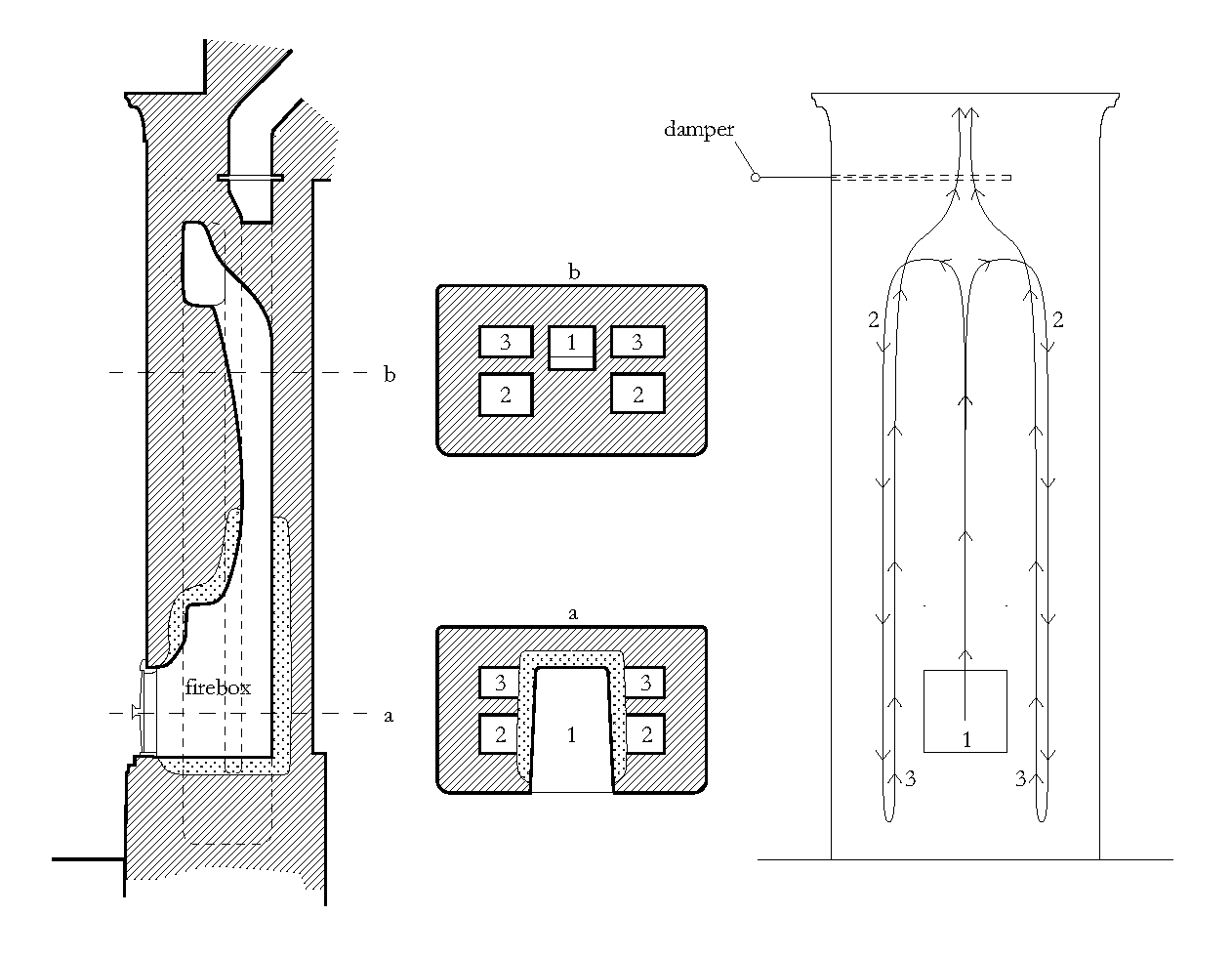
Section, plans, and diagram of a five-channel tiled stove, which was heated not only by the fire but also by the heat contained in the smoke rising from the burning wood. The smoke of the firebox first rises into a narrow duct at the back of the stove (1), then is guided to the front of the stove where it splits into two downward guiding ducts (2), and then at the bottom returns to the back of the stove (3), where it rises up and joins into one duct on the way up to the chimney. The rate of combustion was controlled by sliding the damper. All drawings by author.
With all the labor and responsibility required by wood stoves, it’s easy to understand the appeal of coke-based central heating, both to residents and to real estate developers. Central heating was also the subject of propaganda during the 1910s from entrepreneurs trying to overcome prejudices against the new technology.10 One of the primary arguments for switching from wood stoves to central heating was fuel. Unlike tiled stoves, central boilers could accommodate coke, a new type of fuel that was at many points in early-twentieth-century Helsinki cheaper per joule than firewood.11 While difficult to light, coke gives off highly concentrated heat, and doesn’t waste heat in the form of smoke in the same way that firewood does.12 Coke is also about a third in size relative to its heat value, and, also unlike wood, doesn’t require constant supervision and regular cleaning. Central heating thus offered users the luxury of stable and constant heat while relieving them of the laborious tasks and responsibilities of heating stoves and maintaining fuel supplies. Centrally heated rooms were also thought to require less heating than those heated by a centrally-located wood-fired stove, as radiators could be placed close to the outer walls, thus keeping them drier and improving a brick structure’s insulation capacity.13
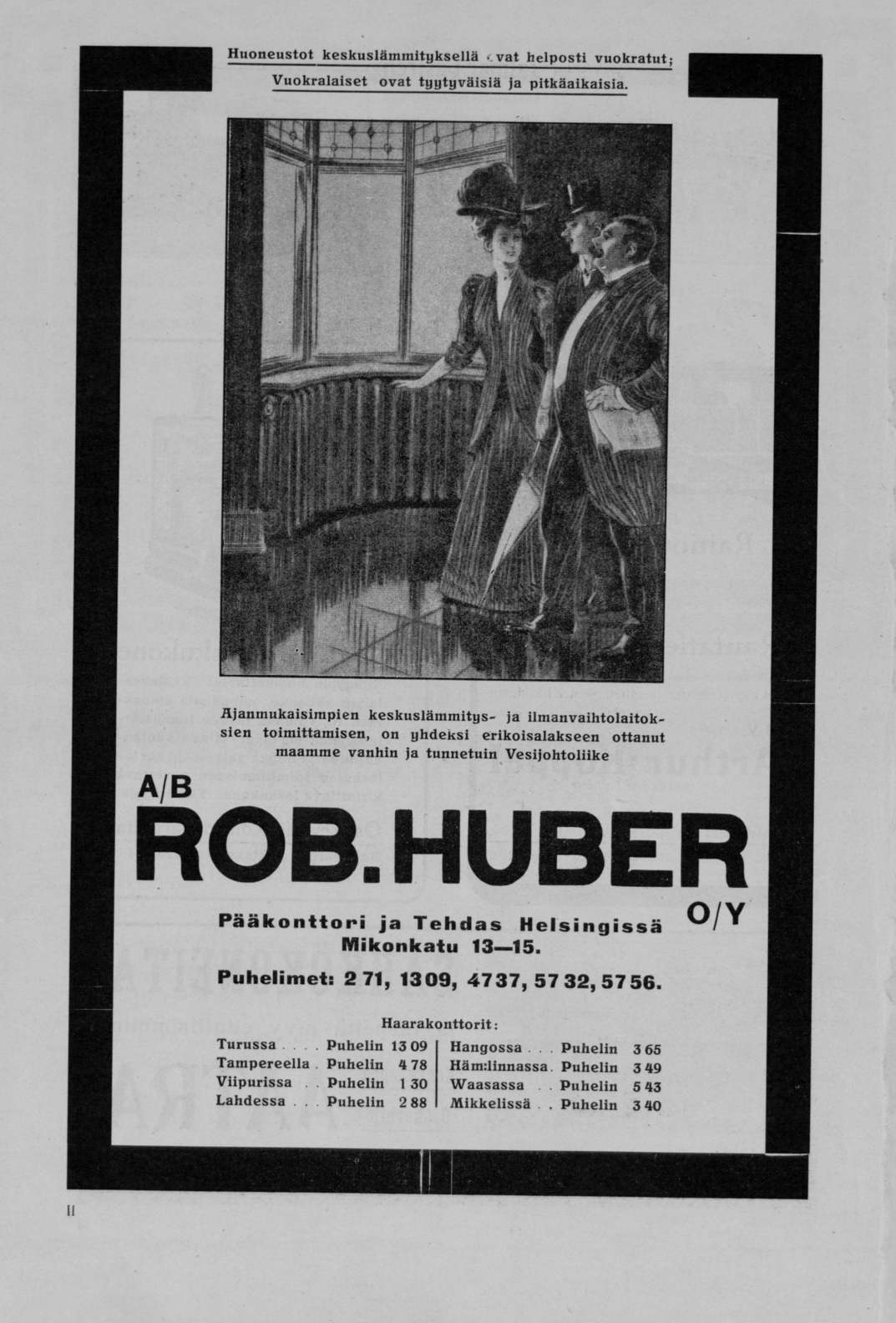
“Centrally heated apartments are easy to rent, and tenants are happy and stay long-term” claims an advertisement by a plumbing business in the January 1912 issue of Teknillinen aikakauslehti. Source: Archive of the National Library of Finland.
With coke-fuelled central heating systems, combustion was detached from the space it heated, and moved to a centralized space, typically in the basement. From there, the groundskeeper would heat the building’s water boilers, which would send hot water to all of the building’s radiators through iron pipes. Heated water was constantly circulated throughout the rooms of the building, and, by adjusting the thermostat, inhabitants could configure the amount of the water going through their radiators.
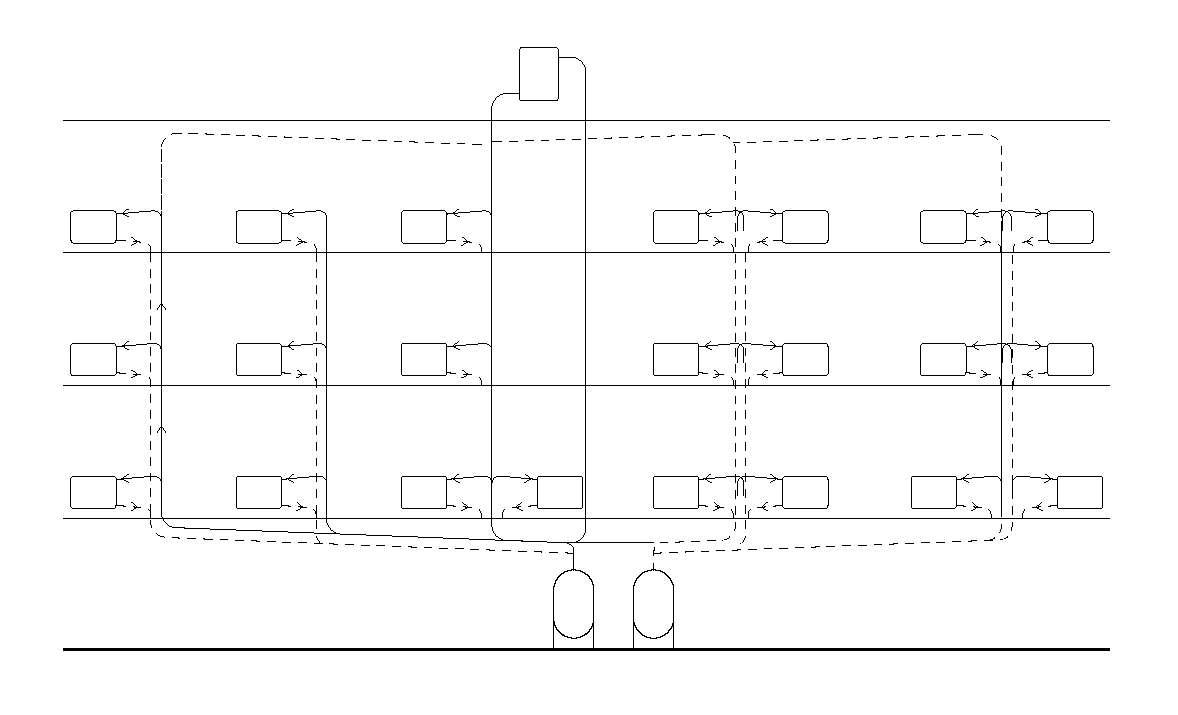
Section diagram a central heating system. Hot water rises from the cellar through pipes connected to radiators, and cooled water runs down back to the boiler. The pipes are connected to an expansion tank at the top of the system (in the attic), which functions as a water pressure equaliser.
With central heating, the act of acquiring fuel was also centralized. Residents were thus no longer exposed to the act of combustion, market conditions, or fuel’s materiality: its dryness, quality, delivery, and stock. Moreover, they were no longer subject to the labor associated with providing comfort; a stark contrast to the nearly instantaneous heat provided by a wood-fired stove being heated. Instead, all of this became abstracted as a cost, which could be divided among residents in proportion to their apartment size—not according to one’s need or amount of time spent in the apartment. Heating just happened.
The shift that started to occur in Helsinki in the beginning of the twentieth century from one form of fuel and heating technology to another brought about complementary shifts in the design of buildings, their floor plans, and what took place within them. One of the architectural consequences of new coke-fuelled central heating systems was an overall reduction in thermal mass and insulation surrounding individual rooms. Masonry stoves and their thick surrounding masonry structures were simply no longer needed. Slabs and walls within the building were also no longer needed to insulate, let alone store heat, as each room was expected to be heated at all times. As a result, buildings came to be designed with thin concrete pillar structures and thin, non-loadbearing partition walls.14 This transition from thick wooden floor slabs to thinner concrete floor slabs in some cases halved a building’s thermal insulation between floors.15
Another difference between the two methods of heating is in the way each impacts a room’s humidity. With a central heating system, the air near the radiator heats up, becomes lighter, and rises. Cooler air then moves in to replace it, moving warm air throughout the room. The radiant heat of the stove, on the other hand, warms objects and surfaces directly. This causes less air to move around the space, and prevents the air from drying out. Higher levels of humidity reduce the body’s ability to lose heat through evaporation. This means that bodies retain heat more effectively and makes cooler indoor temperatures more tolerable. Additionally, the masonry walls adjacent to wood-fired stoves had high heat and moisture retention properties. Essentially acting as buffers, these hygrothermal structures moderated changes in humidity and heat.
The type and quality of heat created by each system is reflected in buildings’ floorplans. Stove heating offered radiant heat in proportion to one’s proximity to the stove and surrounding walls. As a result, it made most sense for circulation to be placed in the coldest parts of the space—as far away as possible from the stove—freeing the area near the stove for everyday activities. As a result, comfort could be achieved by adjusting one’s position in relation to the stove and adjacent brick walls. In contrast, as a source of heat situated close to the outer wall, central heating offered uniform heat throughout a space by countering temperature imbalances. This allowed circulation to be concentrated in the core of the building, and offered space by the outer walls and windows as prime liveable areas. Optimizing comfort with central heating could be achieved by adjusting the thermostat or radiators—at least in principle. It could also be achieved by opening the window.
Wasteful heating habits such as these were noticed as early as 1913. In an article for The Technical Journal, Emil Keso, the then newly-appointed lecturer in heating and ventilation at the Technical University of Helsinki, alluded to the “unlimited waste of heat” common to centrally heated rooms.16 Also around this time, reports began to emerge of centrally heated spaces causing wooden furniture and doors to crack, and plants to die—a consequence of warm air’s circulation. In addition to the significant movement of air throughout the rooms, Keso explained that rooms were being warmed more than they should be, drying them further.17 The historical practice of generating, storing, and depleting heat (and oxygen) had been supplanted by a continuous flow.
In 1925, Keso also noticed that centrally heated rooms had indoor temperatures 3-5°C above those heated by tiled stoves.18 Furthermore, he observed what seems to have been a new form of ventilation: window cooling. Opening the window to cool the space was becoming a common alternative to adjusting the radiator in the winter. During the following two decades, window cooling would consistently be brought up in Finnish public discourse as a problem of central heating’s efficiency. The so-called tragedy of the central heating commons, and the game theory attitude of occupants, was summed up nicely in a newspaper article from 1924:
[Central heating] has its own peculiarities. For the heater, the main thing is to drive coke into the boiler so that the fire has something to eat, whether the fire is heating [radiators] or birds in the sky. For the occupants of the house, on the other hand, it’s all about being very warm; other things are not their concern. If there is too little warmth, the heater is bothered; if there is too much, the windows are opened, and the expensive heat is dumped on the magpies. Few people bother to adjust radiators if there is too much heat, and hardly anyone living in a house with central heating knows that they could adjust their radiators themselves to the right temperature if they only bothered to move the valve a little. While some people take too much heat and let it out of the window, others miss out.19
This critique of central heating seems to reveal a shift in heating behaviour from the era of stove heating. Conceptually, controlling one’s comfort shifted from the actively opting in to heat through the stove, to opting out of heat through the window.
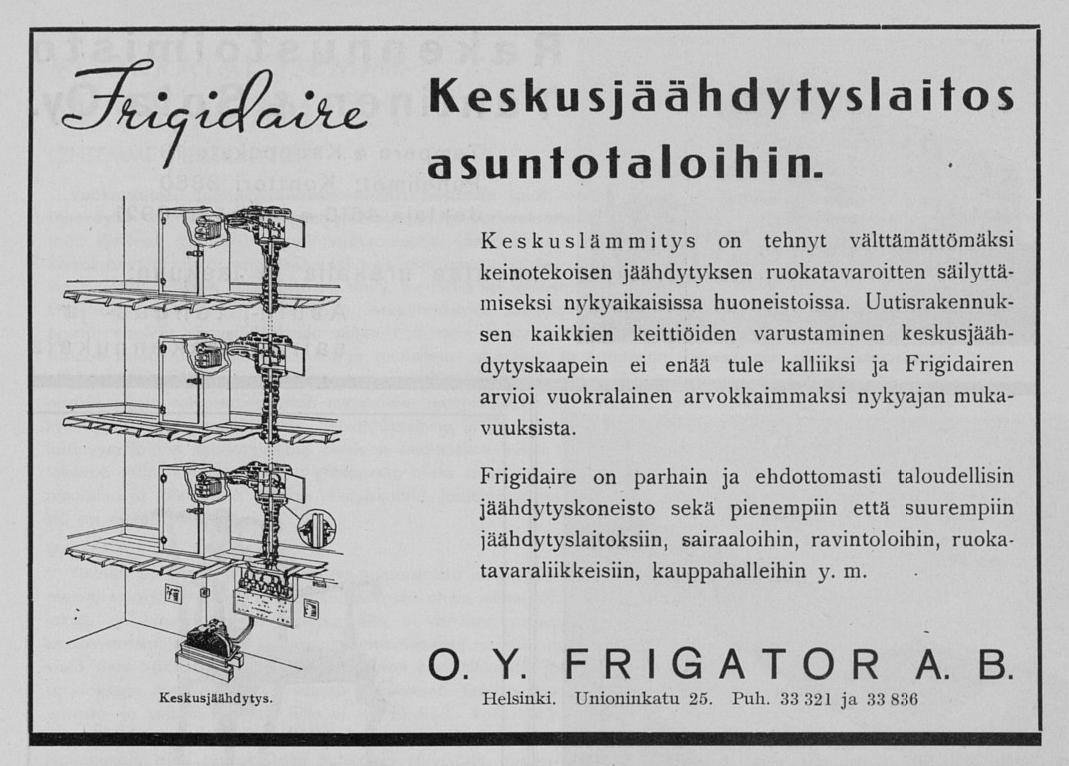
“Central heating has made artificial cooling a necessity for storing food in modern homes,” claims an advertisement for central cooling systems in the February 1935 issue of Arkkitehti. Source: Archive of the National Library of Finland.
What’s next?
Today, the average temperature of a Finnish household is 23°C, while the World Health Organization’s marks the threshold of a healthy winter indoor temperature at 18°C.20 Similar indoor winter temperatures can be found nearby, where a study found that 80% of buildings in Sweden were heated to a temperature higher than 21°C, with one third heated above 23°C.21 Experts say that out of the types of changes needed to meet energy efficiency goals—behavioral, technological, and structural—the behavioral change of reducing winter indoor temperatures has the greatest potential.
While there were many challenges to overcome and limited socio-technical momentum, the history of Helsinki’s transition from wood stoves to central boilers demonstrates the potential for behavioral changes to be induced not in spite of, but with the help of architecture.22 Through design, architecture can both enable and incentivize mindful heating. This is an architecture in which the occupant grasps the amount of energy being used, or one that offers a variety of thermal experiences. The fuel stock in the cellar, the heat of logs in masonry structures, the oxygen in a space, and the body’s warmth trapped by humid air all demonstrate efficient resource use—stored carefully and only stringently released.
Astrid Kander, Paolo Malanima, and Paul Warde, Power to the People: Energy in Europe over the Last Five Centuries (Princeton: Princeton University Press, 2013), 5.
In Finland, the average lifespan of ventilation systems and radiators is estimated at 15 to 25 years. Janne Laksola, “Tiedätkö missä kunnossa taloyhtiösi ilmanvaihto on?,” Kiinteistöliitto Uusimaa (blog), May 22, 2023, ➝.
“Communication from the Commission to the European Parliament, the Council, the European Economic And Social Committee and the Committee of the Regions: An EU Strategy on Heating and Cooling” (2016), ➝.
“EU Parliament Backs Law Aimed at Saving Energy by Renovating Buildings,” Reuters, March 14, 2023, ➝.
Juha Vinha, “The impact of tightening energy efficiency regulations for buildings: Theory and Practice,” presented to the Finnish Parliament, February 11, 2016.
O. Guerra Santin, “Occupant Behaviour in Energy Efficient Dwellings: Evidence of a Rebound Effect,” Journal of Housing and the Built Environment 28, no. 2 (June 2013): 311–27.
See Barnabas Calder’s Architecture: From Prehistory to Climate Emergency (London: Pelican, 2022); and Daniel Barber’s “Heating the Bauhaus: Understanding the History of Architecture in the Context of Energy Policy and Energy Transition” (Kleinman Center For Energy Policy, 2019).
Jan Kunnas and Timo Myllyntaus, “Postponed Leap in Carbon Dioxide Emissions: The Impact of Energy Efficiency, Fuel Choices and Industrial Structure on the Finnish Energy Economy, 1800-2005,” Global Environment 2, no. 3 (January 1, 2009): 177.
In the heating season, one fuelling a day was generally adequate for thermally performing rooms.
See G. Andersson and K. Ekman, Fornnt Och Nytt (Helsinki: Tekniska förening i Finland, 1947), 88.
Strebel was a common brand of central heating boilers.
K.V. Hyvärinen, “Keskuslämmityslaitosten suhteesta uuneihin,” Rakennustaito, November 21, 1925, 275.
Emil Keso, “Uuni- vai keskuslämmityskö? (Stoves or Central Heating?),” Teknillinen aikakauslehti no 4 (1925): 224, ➝.
The masonry walls were still used as passive ventilation channels, as seen in the image.
This is the case in the comparison of Rahapajankatu 1 and Runeberginkatu 35.
Emil Keso, “Keskuslåmmityslaitoksista,” Teknillinen Aikakauslehti 6-7 (June/July 1913), 125, ➝.
“Rakennusmestarien oman kokoustalon hankkimispuuha,” Rakennustaito 8 (April 1916), 106, ➝.
Emil Keso, “Uuni- vai vesilämmityskö?,” Teknillinen Aikakausilehti, n.d., 223.
Translated to English from: Vannas, “Turun kirje,” Uusi Aura 284, October 19, 1924.
Ulla Haverinen-Shaughnessy, “Meneekö sinun asunnossasi helleraja rikki talvellakin?,” THL (blog), 2016, ➝; World Health Organization, “Housing and Health Guidelines” (Geneva: World Health Organization, 2018).
Despoina Teli et al., “Drivers of Winter Indoor Temperatures in Swedish Dwellings: Investigating the Tails of the Distribution,” Building and Environment 202 (September 2021): 108018.
Georgia Savvidou and Björn Nykvist, “Heat Demand in the Swedish Residential Building Stock: Pathways on Demand Reduction Potential Based on Socio-Technical Analysis,” Energy Policy 144 (September 2020): 111679.
After Comfort: A User’s Guide is a project by e-flux Architecture in collaboration with the University of Technology Sydney, the Technical University of Munich, the University of Liverpool, and Transsolar.