We do not grow absolutely, chronologically. We grow sometimes in one dimension, and not in another; unevenly. We grow partially. We are relative. We are mature in one realm, childish in another.
—Anaïs Nin, The Diary of Anaïs Nin1We can only hope that the problem of ancestrality succeeds in waking us from our correlationist slumber.
—Quentin Meillassoux, After Finitude2
In Greenland there are two established species of bumblebees among the relatively low diversity of pollinators, which also includes flies, moths, and butterflies. Bombus polaris and Bombus hyperboreous inhabit the region’s tundra. The bumblebee colonies of Bombus polaris are attacked and enslaved by Bombus hyperboreous, which itself cannot produce workers. This parasitic relationship has likely shaped pollinator coordination across the Arctic tundra for millennia.
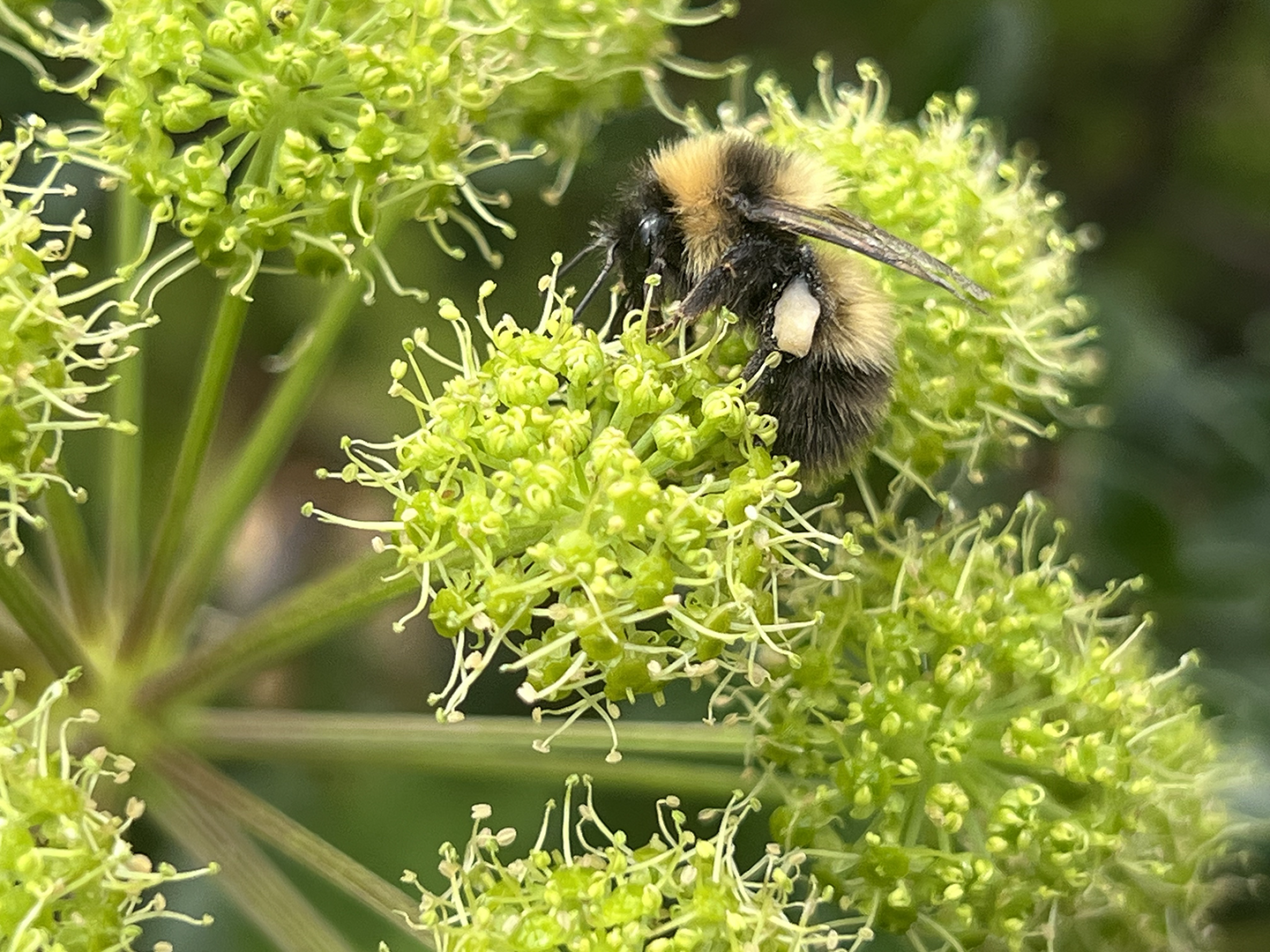
Bombus polaris. Photograph by Brandon S. Whitley.
At the Natural History Museum of Denmark, biodiversity scientists are studying the pollinators of Greenland. The research group, led by Natasha de Vere, has observed that environmental shifts in the Arctic region are not incremental and that the responses from plants and animals to the changes are not gradual. The seasonal patterns in Greenland are becoming increasingly unpredictable due to growing environmental stochasticity, or randomness.3 During summers, when researchers gather pollinators for study, the environmental volatility is most evident. In one year, the snow on the tundra may not melt until June, delaying the pollinator season, while the next year, it might melt much earlier, causing the flowering season to begin and end early.4
Knowledge of these shifts highlights the significance of contemporary scientific methods, such as using pollen DNA metabarcoding to identify the plants that pollinators visit.5 By swiping pollen from a batch of seven to eight hundred collected pollinators, scientists can trace which plants have been visited by which insect specimens. Using supercomputers, they then match the DNA extracted from the pollen with plant DNA databases. This sequence of steps, from field collection in summer to DNA analysis, takes around two to three months. The results provide “behavioral snapshots” of pollination interactions from the day researchers capture the insects, offering insights into how pollinator-plant relationships change over time. The scientists performing these studies say that what they see looks more like unpredictable chaos than conscious migration and adaptation.
The influx of new data frequently outpaces and even contradicts historical records, revealing patterns and interactions that were previously undocumented or thought highly improbable or impossible. For instance, non-native species that are adapted to warmer climates are now venturing into the Arctic, which was previously unthinkable. As the Arctic climate moves rapidly away from historical baselines, traditional ecological theories—often built on limited, linear datasets—are also destabilized (for instance, models have assumed a consistent blooming and pollinator cycle that new data shows is now often mismatched as temperatures rise).6 According to Natasha de Vere and her group, this points to a wider story about our current abilities to understand the history and future of nature: “As volatile environmental shifts overtake our traditional baselines, we’re facing the reality that historical data offers only a partial lens through which to interpret the chaotic, nonlinear changes before us.”7
Consequently, analytical models designed for smaller or more stable data sets are stretched beyond their limits, often unable to account for chaotic variability and rapid shifts in species behaviors and interactions (such as erratic pollinator activity due to rapid freeze-thaw cycles, which drastically impact species survival and pollination success). This “data deluge” is pushing researchers to reevaluate their understanding of Arctic ecosystems, especially in a world where adapting to rapid, unpredictable environmental changes is crucial. De Vere captures the enormity of this challenge, observing that “as our analytical approaches develop, we see an ever-expanding fractal landscape of unknowability revealing itself.”
Wall of Complexity
This flood of new, contradictory data reveals the underdetermination of science. Underdetermination is the inability of evidence alone to provide a unique explanation for events. This is distinct from “undetermined,” where knowledge is inaccessible, or “overdetermined,” where multiple causes lead to the same result. Instead, underdetermination arises when scientists must navigate systems where evidence often leads to multiple plausible explanations, requiring interpretation through theoretical frameworks and assumptions. More data and scaled-up tools can generate stable affirmative scientific results, even as they fuel destabilizing tensions within science. For instance, the observations of new pollinator interactions in shifting Arctic landscapes force scientists towards interpretive leaps that both enable insights into ecosystemic shifts and expose the limits of existing ecological models. What is profound today are the intertwined challenges of epistemological breakthroughs—which lead to overwhelming complexity in knowledge production—and radical environmental changes that push living processes into uncertain and unpredictable dynamics. This reflects the enduring reality that life’s complexity surpasses human comprehension, and is constrained by the perspective of human experience.
Biological sciences are at the beginning of a substantial rethinking of whether it is possible to know how life works, a tension that has shaped the field since its inception. Scientific epistemologies are reaching “walls of complexity” due to the accessibility of increasingly granular scales, global data capture, and computational modeling possibilities.8 What has been termed a “complexity crisis,” or “peak complexity,” suggests that the biological sciences are undergoing an epistemological shift towards embracing approaches that try to account for life’s inherent unpredictability, relationality, and emergent dynamics.9 This makes it very hard to hold a firm scientific grip on biological reality, and makes it increasingly difficult to believe humans can fully answer basic questions across the scales of life, such as: How does biodiversity change with the climate? What causes cancer? What lives in the human microbiome?
I arrived at these questions through working with strategic development initiatives and artistic projects at natural history museums and natural science institutions. I became interested in what happens when the biological sciences approach new thresholds of complexity, and when progress in basic research brings us closer to seemingly unanswerable questions. This essay is written through dialogues with scientists from natural history, microbiome science, and cancer research, who recognize the significance of these complexities in their work. In this valley of underdetermination, scientific technologies and models not only provide answers but also increasingly expose the expanding scope of what remains beyond our understanding. It is time to reflect on the implications of this spreading axiomatic crisis.
What Does Your Gut Say?
Since the 1970s, scientists have estimated that the number of microbial cells containing nonhuman DNA in and on the human body is roughly equal to the number of human cells that make up the entire body.10 Initially, microbiome research relied on culture-based methods, which only identified a limited subset of microbial species. However, the advent of high-throughput sequencing and metagenomic analysis in the 2010s allowed researchers to uncover thousands of previously undetectable microbes, revealing that the human microbiome is a dynamic ecosystem that changes in response to diet, environment, and lifestyle.11
The human gut, hosting the body’s most diverse microbial ecosystem with an estimated ten to forty trillion cells, has since become a focal point in microbiome studies. The diverse populations of microbes in the gut reflects a wide variety of human factors, including early life stages, diet, sleep patterns, climate, and surrounding organisms. These influences have shifted microbiome research from a focus on individual health—such as the role of gut microbiota in digestion and immunity—to a broader view that links microbiome health to societal and environmental factors like industrialized diets, antibiotic use, and pollution. Researchers now acknowledge the microbiome as both a personal and collective phenomenon, linking individual wellness to ecological and public health. Originally regarded as a quasi-stable “second genome” or genetic counterpart to our human genome, the microbiome is now understood as a plastic system capable of adapting dynamically to its host’s environment and habits while maintaining quasi-stable states. Its species boundaries are fluid, with bacterial colonies capable of rapid genetic adaptation through processes like horizontal gene transfer. This evolving genetic landscape challenges traditional taxonomies and requires researchers to adopt multidimensional, dynamic approaches to study it.
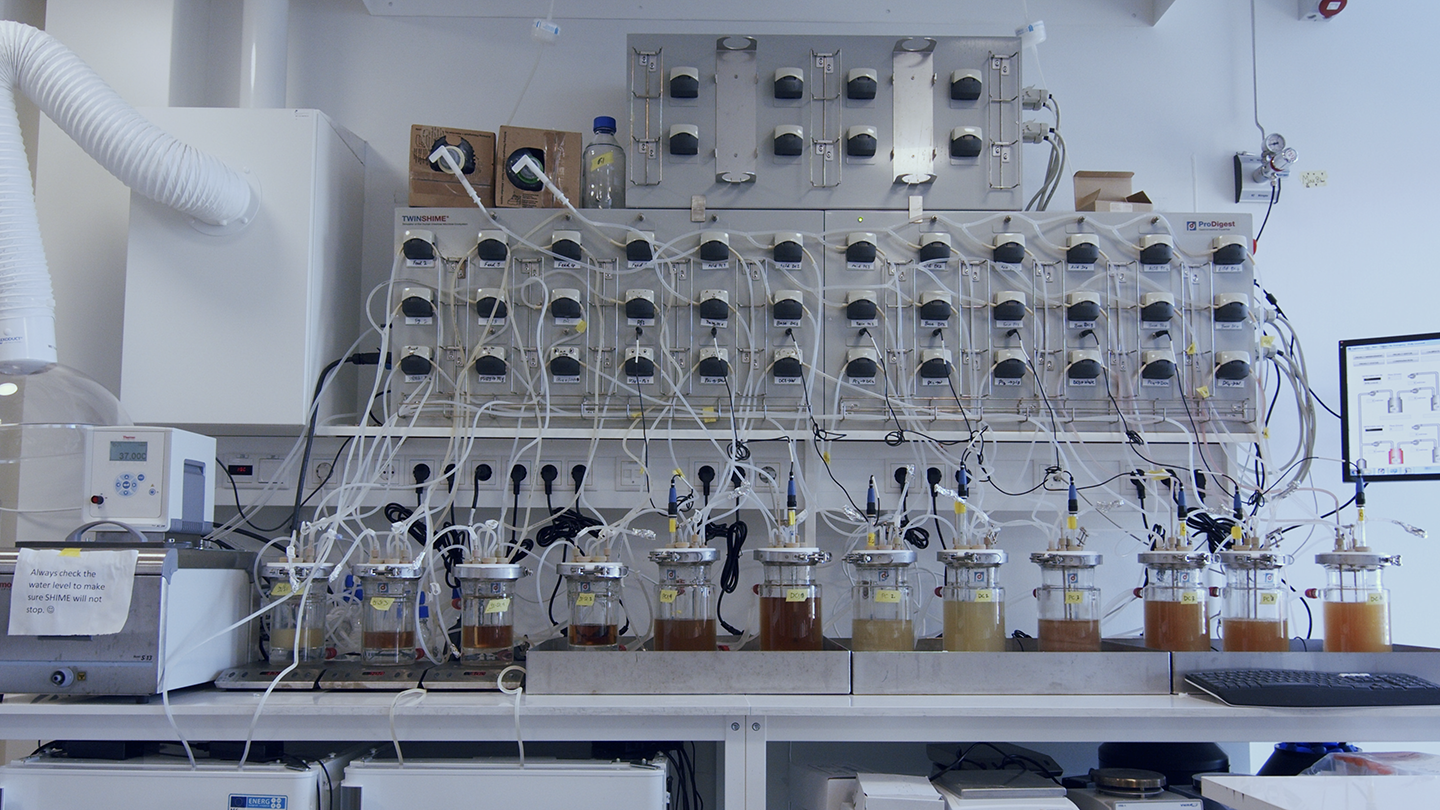
Still image from Jenna Sutela’s 2022 film Milky Ways, featuring a digestion simulator (SHIME) at the CBMR laboratory in Copenhagen.
At the Novo Nordisk Center for Basic Metabolic Research (CBMR) in Copenhagen, scientists use advanced tools, such as the Simulator of the Human Intestinal Microbial Ecosystem (SHIME), to study gut microbiota in vitro. Developed in 1993, the SHIME mimics the human gastrointestinal tract, allowing researchers to observe changes in microbiota during digestion without invasive sampling.12 My initial encounter with SHIME came in 2020, during a collaborative film project with colleagues Jenna Sutela, Mandus Ridefelt, and Adam Bencard initiated through the platform Primer. Working closely with the lab’s systems biologists, this partnership evolved into a longer interdisciplinary research process involving Mandus Ridefelt, scientists at CBMR, collaborators from Lund Cancer Center, and myself.
Led by Mani Arumugam, the CBMR team uses advanced sequencing to explore microbial diversity at the species level, capturing real-time genetic mutations and evolutionary dynamics. This advanced sequencing approach highlights the fluidity of microbial populations and shows that species definitions within the microbiome are constantly shifting, reflecting its adaptability and variation among individuals. Such a real-time view of evolutionary dynamics highlights a rapid process in which species definitions can change based on the thresholds established by advanced bioinformatic tools. This evolving genetic landscape indicates that species boundaries within the human microbiome are not stable, suggesting that diverse microbial populations can vary significantly between individuals. As a result, researchers may discover new microbial species or variations every time a new individual is sampled.
Arumugam and his team employ higher-resolution sequencing that questions established benchmarks for species definitions within the microbiome. The rate of genetic mutations and adaptations they encounter often outpaces their analytical capabilities, creating a dynamic landscape where new microbial variations emerge faster and more broadly than they can be catalogued or fully understood. Yet this accelerated view into microbial dynamics is also a window into ancient relationships, stretching back millions of years. As Arumugam puts it, “There is plenty of evidence to conclude that gut bacteria predate the human lineage. We’ve had bacteria in our gut before we became humans and gut bacteria existed within the ancestral species that eventually gave rise to mammals.”13 Before mammals and birds diverged, their common ancestor harbored a single type of gut bacterium—an ancestral microbe that would later give rise to both E. coli and salmonella. As mammals and birds evolved separately, this bacterium coevolved with its hosts, eventually diverging into the distinct species we recognize today. This extensive evolutionary history positions the dynamic properties of the microbiome as a living link between current human biology and deep evolutionary time.
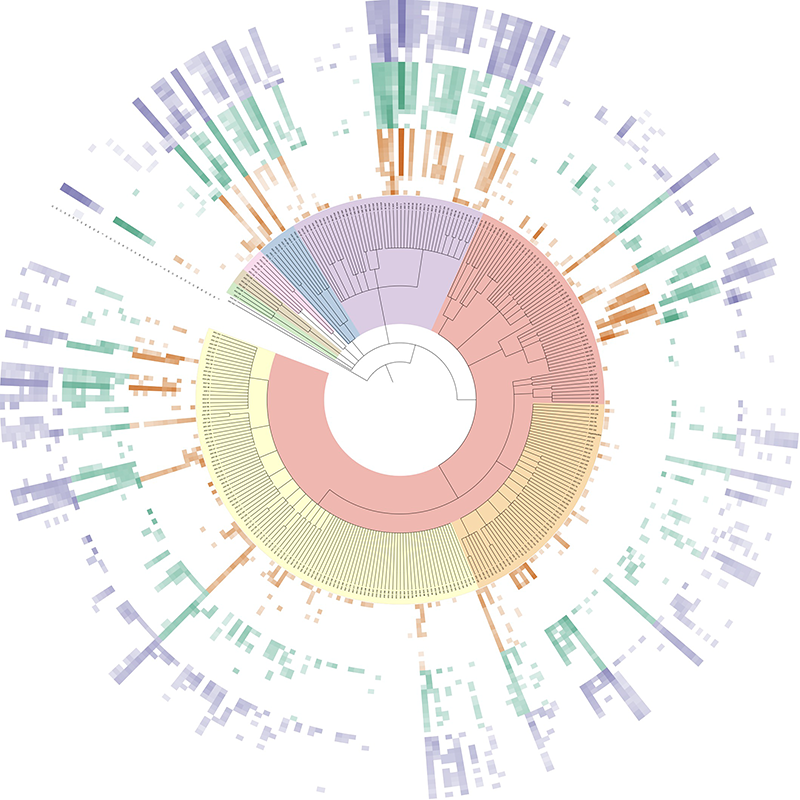
This 2024 visualization from the Arumugam Lab at CBMR tracks changes in microbial populations within SHIME over a month. The colored phylogenetic tree indicates possible bacterial affiliations, while the concentric circles depict their relative abundances. The absence of certain bacteria in earlier periods, with their appearance later, highlights both detection uncertainties and shifts in microbial composition.
Estimates by researchers in the lab suggest that around 20 percent of the gut microbiome is well-characterized, while approximately 40 percent is actively studied. Another 40 percent remains largely uncharacterized, limited by the dynamic and ever-changing properties of the microbiome and the constraints of current classification technologies. Researchers in the lab acknowledge that each breakthrough not only introduces new layers of complexity but also multiplies the scientific questions that remain, and may continue to remain, unanswered. Their research does not tip the scale towards overall clarity but rather adds new layers of complexity, surpassing their ability to validate existing frameworks and accelerating underdetermination. Arumugam reflects on this paradox, noting that “as long as we are finding more and more answers to questions, we will enlarge a black hole of unanswered questions in the middle of our discipline.” The evolving trajectory of gut-microbiome research—where more knowledge amplifies uncertainty—reflects a broader shift in metabolic sciences that embraces the unsettling possibility that some questions around metabolic regulation and disease origins may remain beyond our grasp.
What Causes Cancer?
From the complex interconnections of the microbiome to the intricate workings of individual cells, cancer research reveals a comparable depth of uncertainty, where genetic mutations destabilize the very systems that sustain life and amplify unresolved questions about cellular regulation and biological stability.
Cancer arises from genetic mutations that disrupt normal cell regulation. Cancer is often viewed as a byproduct of complex life forms, raising ongoing questions about its role as either a natural biological occurrence or a pathological aberration (or both). Once thought to be caused by trauma or irritation, cancer began to be understood as a disease of genetic malfunction by the mid-1900s. The discovery of oncogenes in the 1970s—genes that drive uncontrolled cell growth when mutated—accelerated this shift. Cancer was likened to a biological “code error” initiated by single-cell mutations.
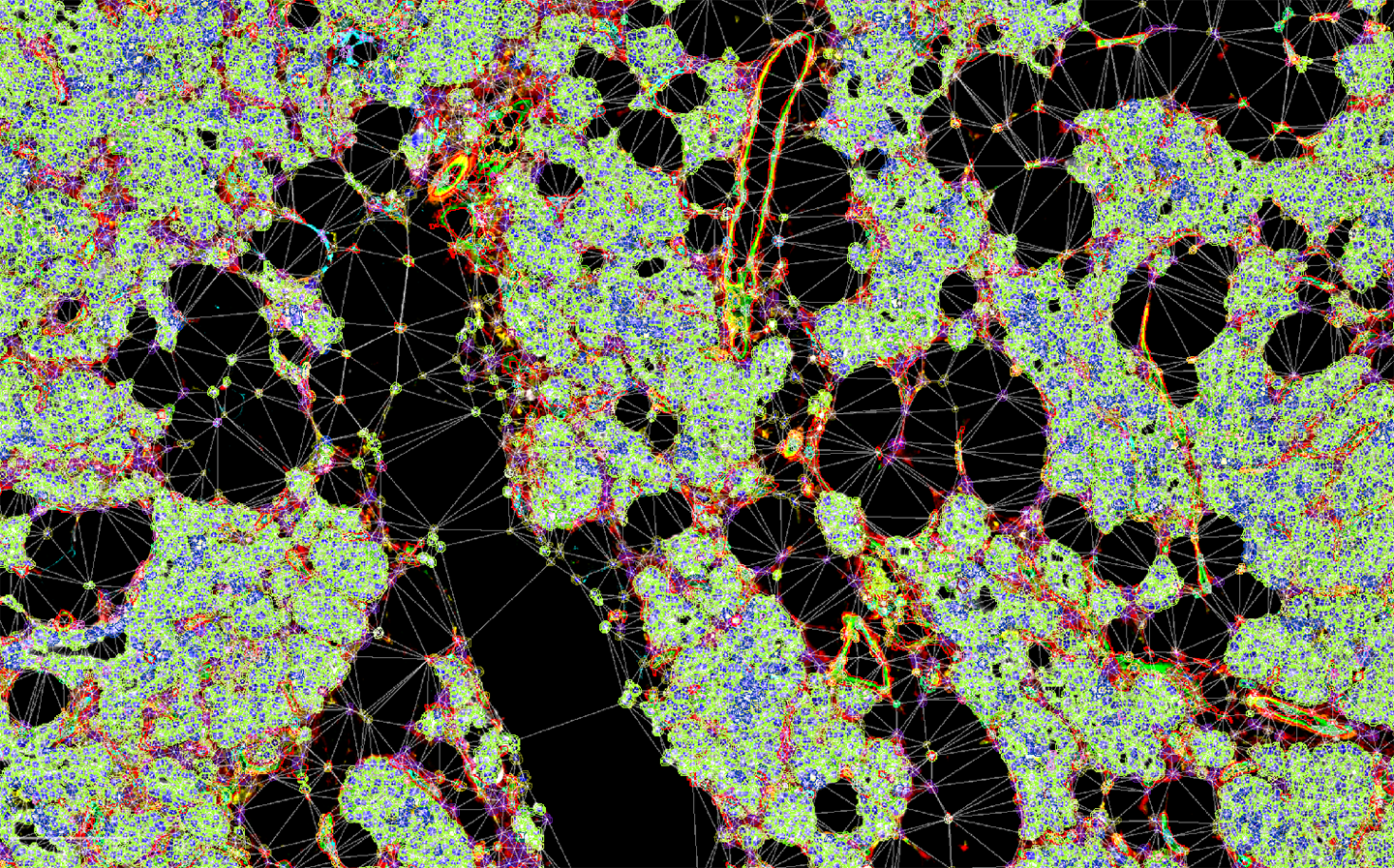
Visualization of cancer tumor connectivity: spatial relationships and interactions between diverse cell types within a tumor microenvironment. Image by Jessica Pantaleo.
However, today’s cancer science operates within a post-genomic framework, which acknowledges that genetics alone cannot fully explain cancer. Advances in data collection, particularly the rise of single-cell sequencing, have propelled this shift, making bioinformatics and machine learning essential for analyzing the vast, complex datasets generated by modern cancer research.14 This broader perspective incorporates various -omics and -etics fields, including transcriptomics (the study of RNA molecules that help turn genes into proteins), proteomics (which examines the entire set of proteins produced by cells), and epigenetics (which looks at heritable modifications in gene expression that do not alter the DNA sequence). In the post-genomic framework, cancer is a perturbation in the organism’s systemic relations, extending beyond isolated issues to disrupt broader biological functioning. Questions about cancer’s causal structure merge with wider questions of how life’s forms continually emerge from life’s own dynamic processes.
At Lund University Cancer Center, the Translational Cancer Lab led by Kristian Pietras is piloting research in another post-genomic trajectory: the tumor microenvironment. In the 1980s this field of cancer research focused on how tumors attract blood vessels, with researchers attempting to block this process by using various treatments. By the 1990s, molecular targets were found, leading to anti-angiogenic drugs like Avastin. Launched with a mix of scientific optimism and aggressive marketing in the 2000s, these treatments had limited success in extending survival of cancer patients and caused significant side effects, shifting research towards the broader, complex tumor-microenvironment relationship.15 Today, the Lund lab focuses on various dimensions of the microenvironment, including immune responses, blood vessel formation, and supporting cells like fibroblasts, which provide structural support and help maintain the extracellular matrix.16 Tumor heterogeneity (the genetic diversity within a single tumor) and tumor evolution (the changes that occur within tumors over time) are also crucial factors in cancer progression.
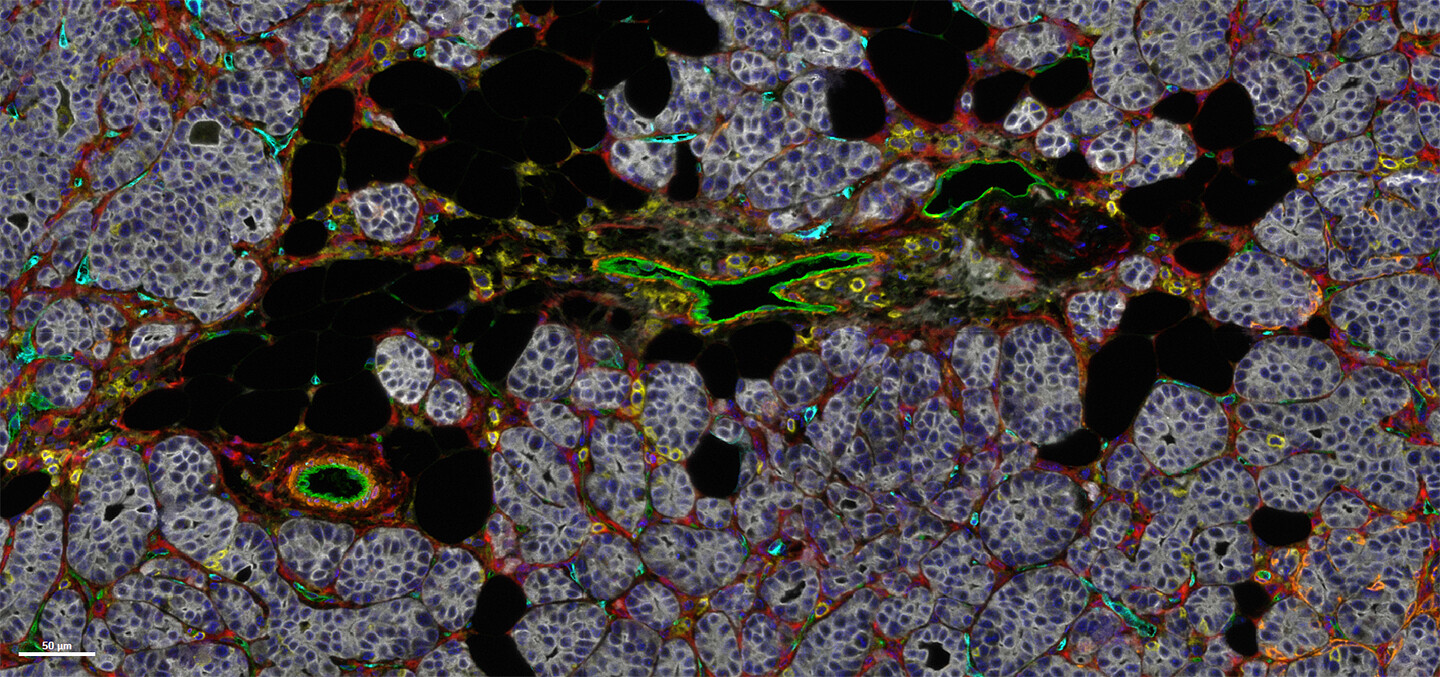
Visualization of the tumor microenvironment in experimental breast cancer: highlighting interactions between White –tumor cells, Green – blood vessels, Orange –smooth muscle cells,Yellow –immune cells, Red –connective tissue cells providing structure, and Turquoise –surrounding blood vessels.
Researchers sense a drastic shift coming thanks to the availability of large-scale cancer sequencing data and the increasing use of analytical models that encompass larger patient groups and more variables. Statistical inference methods now dominate research, but they often bypass functional validation. This critical step involves testing hypotheses in systems like in vitro cell cultures or in vivo organisms to ensure they hold true under the full complexity of real biological conditions. While surges in data and complex analytical possibilities feed the ambitions of individualized medicine—like RNA vaccines—the increase also complicates our understanding of phenomena such as cancer, making it seemingly impossible to find clear pathways in a dense forest of information. Pietras captured this complexity when he stated, “You get very dizzy if you try to understand what the tumor is. What we thought we knew a decade ago now seems like a simplified view in light of what we can understand today.”
The most common form of colon cancer typically develops from stem cells located at the bottom of colonic crypts (there are approximately one hundred crypts per square millimeter across the colon’s 160-centimeter epithelial surface). In-depth research on mutations within these crypts shows that many cells carry mutations but only a small fraction lead to the formation of cancerous tumors. Part of the mystery of the research is that the mutational patterns in colonic samples from patients without cancer look identical to those in cancer patients, but no tumors develop. This ambiguity raises questions about the environmental factors involved, and whether these mutations will trigger cancer or remain dormant. Researchers are not only uncertain about how cancer develops and progresses; they also don’t know why, despite the presence of mutations commonly associated with cancer, it sometimes doesn’t begin at all. This ambiguity exemplifies underdetermination: the same mutational evidence can point to multiple plausible pathways, none of which can be definitively confirmed.
It is challenging to ascertain whether cancer begins at a single moment or is an intrinsic part of life’s cellular systems, emerging from their inherent complexity. This ambiguity reflects a recurring historical pattern in science, where questions about evidence and complexity push the boundaries of established frameworks.
We’ve Been Here Before
It is crucial to emphasize that underdetermination is inherent in science’s dialectical nature, which involves an interplay between discovery, analysis, and critical examination. Throughout history, pressures on axiomatic systems—which frame the logic of knowledge production—continuously push the boundaries of scientific questioning outside its own comfort zones. To ground this inquiry, I look to mathematician Bonnie Shulman, whose critical work in the history and philosophy of mathematics has been largely overlooked. During the 1970s, before Shulman started her scientific career, she worked with poets Allen Ginsberg and Anne Waldman at the Naropa Institute’s Jack Kerouac School of Disembodied Poetics. Through her five years there (transcribing handwritten journals and audio recordings from the Talking Poetics series), she developed a spirit of cultural critique and resistance to conventional boundaries that carried over to her approach to mathematics.
In her 1996 paper “What If We Change Our Axioms? A Feminist Inquiry into the Foundations of Mathematics,” Shulman argues that mathematics, often seen as objective, is deeply shaped by Western cultural values.17 She shows how Western axiom-based reasoning reinforces binary, hierarchical structures, favoring exclusive truths over pluralistic, relational perspectives. For instance, Western reliance on the law of the excluded middle—asserting that statements must be either true or false—contrasts sharply with Indian logical frameworks, where proofs aim to communicate understanding rather than enforce agreement, creating space for multi-valued perspectives.18 Similarly, Chinese linguistic structures encourage seeing objects as dynamic and interconnected processes underscoring a worldview focused on relationships rather than fixed categories.19 Although mathematical language strives to exclude subjective values, Shulman reveals that Western cultural ideals, including those coded as masculine—such as control, hierarchy, and abstraction—have historically been embedded within its logic. These values shape the discipline’s framework, constraining its openness to alternative interpretations and narrowing its capacity to engage with more fluid and inclusive approaches.
This 1996 article was her first and only published feminist critique of mathematics and it faced strong resistance at the time. Her work was met with hostility from scientific colleagues (some labelled her a race traitor) and her tenure at Bates College was initially denied partially on the grounds of the paper. While many feminist scholars critiqued the assumptions underlying various scientific fields, few approached mathematics from within as practitioners. Shulman’s work, though contributing to these critical movements, was left without institutional support or a professional community. With the help of colleagues, she appealed and eventually received tenure, but she then shifted her career focus, teaching the history of mathematics and engaging her students in the politics of gender, class, and race but never publishing on gender in mathematics again. Shulman’s article explores three critical moments in Western mathematics where foundational beliefs about certainty were challenged and redefined.
Three Crises of Certainty in Western Mathematics
Shulman’s first case study shows how irrational numbers contradicted the Pythagorean ideal that all numbers can be expressed as simple ratios, like 1/2 or 3/4. In the fifth century BCE, the discovery of irrational numbers like √2 shattered the Pythagorean belief in a universe governed by harmony and rationality. This realization challenged the notion of logical certainty and introduced philosophical tension into Greek thought, raising questions about the nature of mathematical truth and the limitations of human understanding. This crisis ultimately led to reevaluations within Greek mathematics, inspiring figures like Eudoxus (circa 390–340 BCE) to develop more flexible, axiomatic approaches that could incorporate such contradictions.
The second crisis analyzed by Shulman emerged in the late seventeenth century, following the independent development of calculus by Newton and Leibniz, a shift rooted in paradoxes dating back to Zeno’s 450 BCE inquiries into motion and continuity. The advent of calculus introduced an array of new concepts, most notably infinitesimals—quantities so small that they defied conventional logic. Calculus was quickly embraced and applied widely across mathematics, physics, economics, and politics, often without considering its theoretical foundations. Calculus allowed mathematicians to analyze phenomena—such as motion and acceleration, orbital mechanics, and fluid dynamics—that had previously been impossible or difficult to quantify. However, this newfound power came with complications, as contradictions emerged that exposed foundational issues within the mathematical framework. Infinitesimals were applied intuitively, without rigorous definition, and relied on levels of abstraction that ultimately led to ambiguity and confusion. This uncertainty in turn led to a fundamental reevaluation of mathematical analysis; its foundations were reshaped to address the contradictions calculus had revealed.
Shulman’s third case study, set theory, emerged in the late nineteenth and early twentieth centuries, raising fundamental questions about the nature of infinity and continuity. Georg Cantor (1845–1918) introduced transfinite numbers by distinguishing between countable infinities (like whole numbers) and uncountable infinities (like points on a line), establishing a foundation for modern set theory. His view of infinity was shaped by Aquinas’s division of reality into the finite (comprehensible to humans) and the infinite (reserved for God). Cantor, however, extended this by proposing that some infinities—what he called transfinite sets—were within the realm of human comprehension, while other infinities—what he called paradoxical sets, like the set of all sets—symbolized the Absolute Infinite, a concept beyond human comprehension. These paradoxical sets, being multiplicities, never achieve the unity needed to become individual objects, thus embodying a philosophical paradox between unity and multiplicity. Cantor’s work initially appeared to resolve this paradox, but subsequent contradictions, such as Russell’s Paradox (demonstrating that “the set of all sets that do not contain themselves” cannot consistently exist), shook the foundations of mathematic by shattering the belief in a single concept of infinity.
These case studies lead Shulman to conclude that, despite repeated challenges to the two-thousand-year-old reliance on Euclidean geometry, which is built on axioms seen as self-evident truths, “mathematicians never gave up or questioned the belief that there was some ultimate foundation for absolute truth.”20 This tension echoes the problem formulated by Quentin Meillassoux as “correlationism” in his book After Finitude—the view that we can only understand the relationship between thought and reality, never absolute reality itself as it exists independently of human perception. The historical challenges of Euclidean geometry as an unquestioned system leads us to ask: If axioms are choices rather than absolutes, what gives them authority as truth-bearers?
Mathematicians have historically relied on intrinsic justifications (principles seen as self-evident) and extrinsic, pragmatic reasons to support their choice of axioms. As Shulman suggests, this reflection opens a deeper inquiry: “If our axioms do not derive their truth value as idealizations of physical reality, the question still remains: what criteria shall we use to choose our axioms?” In questioning these foundations, she reveals science not merely as a system for understanding the world, but as a reflection of our cognitive assumptions and limitations. In this vein, a scientific crisis is an opportunity to open up space for asking where our ways of thinking scientifically stand in the world. What does science do, and what is it used for? And how do we see ourselves through it? Shulman concludes that “when we self-consciously reflect on the framework in which we view the world, we are shown a picture of our own cognitive processes. And looking in this mirror, we meet ourselves.”21
How to Stay Perplexed
Turning this mirror back towards contemporary biological sciences, Arumugam of CBMR offers a deeper perspective on his role as a systems biologist: “We are but a speck in the world, trying to observe something vastly beyond our grasp with tools that are increasingly sophisticated yet fundamentally limited. Whatever stories we create, ultimately we are not at the center, and there is no single story about ourselves.” His insight suggests a growing awareness within science that the complexity of life often weaves together scientific understanding with existential questions.
The advanced tools used to sequence, model, and analyze biological data provide unprecedented insight into the intricacies of life, yet they also reveal the boundaries of mechanistic certainty. As more aspects of the biological world become “datafiable,” vast amounts of information resist neat conclusions. Knowledge spills beyond singular narratives, challenging the models meant to contain it. Within these gaps—where data resists synthesis and certainty appears layered—it becomes necessary to ask what it means to know, and how human understanding fits into a universe that may never fully reveal itself.
It is within this space of uncertainty that existential dimensions begin to surface. The deeper the understanding of life’s complexity, the clearer the limitations of that understanding appear, suggesting a potential for something inherently transcendent in life itself—something beyond what data and models alone can convey. This reflection mirrors the longstanding relationship between science and the cosmic unknown, where questions about existence have always lingered. Pietras, reflecting on his work in oncology, observes: “Astronomy has always encompassed more than just science; it raises existential questions inherent to the field. Perhaps we are beginning to witness a similar phenomenon, but at the microscopic scale.” This uncertainty parallels the mysteries of astronomy, such as theories about what existed before the Big Bang or what lies beyond the observable universe. As Pietras observes, the biomedical sciences present analogous challenges, albeit on a microscopic scale. Like the macroscope, the microscope does not solely deliver certainty but exposes layers of complexity that evade explanation. Here, the microscopic world becomes a frontier for both scientific inquiry and existential reflection—a place to explore the profound mystery at the intersection of life, consciousness, and the unknown.
This planetary view is linked to Shulman’s insights into foundational crises in mathematics, where questioning absolute truths opened new directions in understanding. Likewise, the rapid expansion of the biological sciences today reveals that knowledge and unknowability are woven across various scales. Scientific engagement thus becomes more than an attempt to model the living world; it is a continuous process where life, in all its forms, reflects upon its own nature. Here, boundaries of certainty expand into boundless possibilities of meaning, inviting an encounter with life’s deeper mysteries—a kind of rationality that extends beyond any single species, including humanity itself.
What Comes Next?
Canaanite religion (circa 3300–500 BCE in the Eastern Mediterranean) is most known for its polytheistic beliefs and practices, centered on a pantheon of gods and goddesses associated with life, nature, and fertility. This pantheon included Baal, the storm and fertility god; Asherah, a mother goddess tied to fertility; El, the creator deity and chief god; Anat, a fierce goddess of war and love; and Mot, the god of death representing the underworld. Canaanite religious practices were tied to the agricultural calendar and included communal gatherings, feasting, and ceremonies aimed at invoking divine favor for bountiful harvests. The Canaanite religious calendar was deeply syncretic, absorbing elements from neighboring cultures. This created a tapestry of beliefs that influenced other ancient Near Eastern religions and later shaped Hebrew, Phoenician, and Carthaginian traditions.
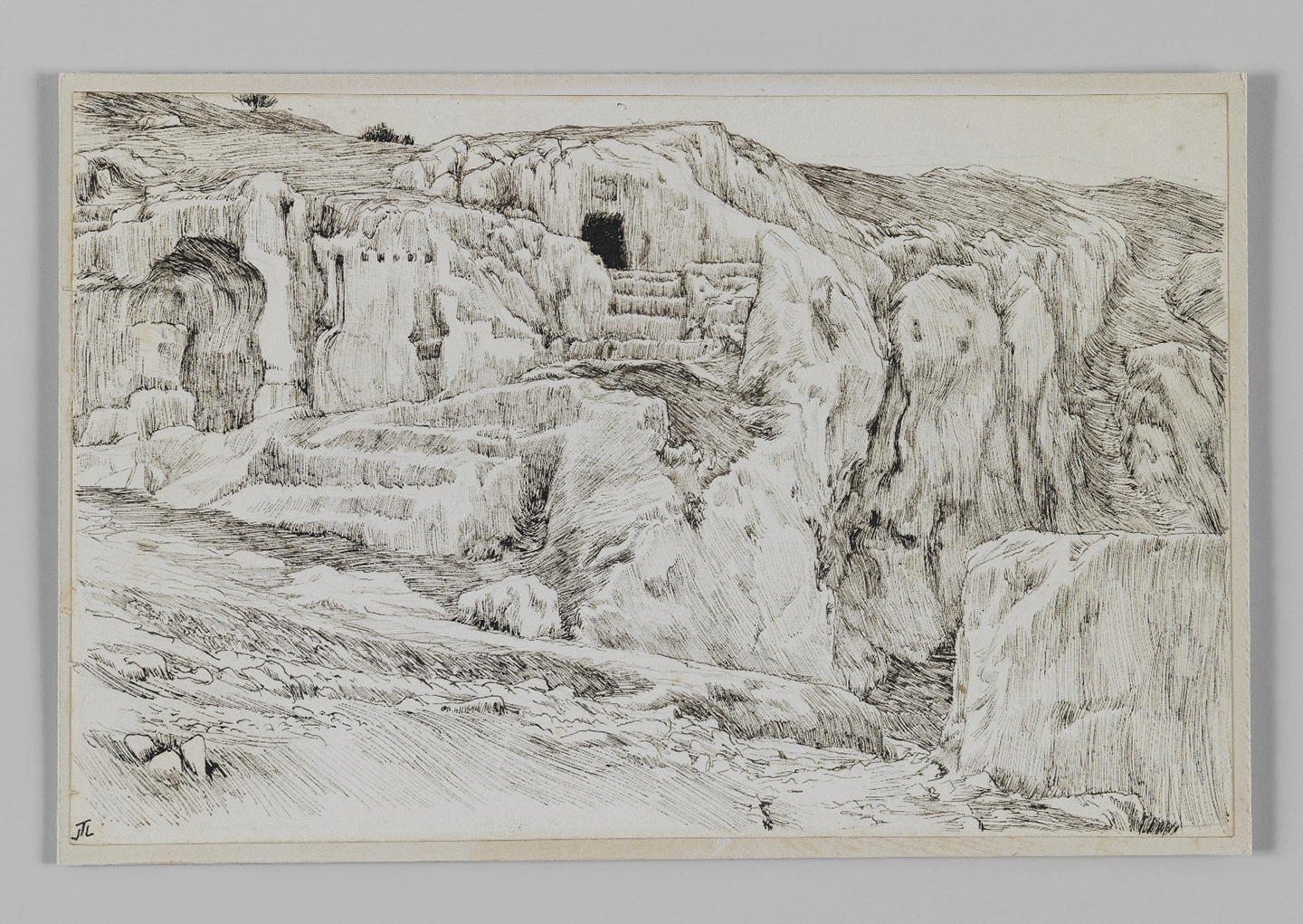
James Tissot, Ancient Tombs, Valley of Hinnom, 1886–1889. Pen and ink on paper. This artwork depicts the Valley of Hinnom, a site historically associated with Biblical references to child sacrifice and the term Tophet. While geographically distinct, the term Tophet has also been applied to describe ritual burial grounds in Carthaginian and Phoenician contexts. Collection of the Brooklyn Museum, purchased by public subscription. Image courtesy of the Brooklyn Museum: 00.159.400.
One of the most infamous aspects of Canaanite religion is the figure of Moloch. Historical accounts, particularly from Greek and Roman sources, suggest that some Canaanites or Carthaginians believed that burning children as a sacrifice to Moloch could bring favor in times of crisis or war. In this ritual, participants sacrificed the most literal sign of the future to appease a deity and gain advantages. Archaeological evidence from the Tophet (a burial site associated with ritual practices) in Carthage includes cremated remains of children, animal relics, and inscribed stelae often linked to ritual practices. While many scholars interpret this as evidence of child sacrifice, others suggest it reflects burial traditions for children who died naturally. This ambiguity underscores the complexities of interpreting archaeological evidence, where material findings suggest possibilities without fully resolving historical debates.
Regardless, the myth of Moloch has echoed throughout history, shaping perceptions of ancient societies. Retelling this kind of gruesome myth has no doubt served the purpose historically of dehumanizing one’s enemy, which is why many scholars caution that such accounts may have been used as propaganda. In the fifth century BCE, Herodotus characterized the sacrifices of the Carthaginians as barbaric, creating a contrast with the rationality of Greek culture, though without specific reference to Moloch. In the late Roman Republic, Cicero broadly condemned child sacrifice, employing Moloch as a symbol of moral decay to advocate for virtuous governance. Early Christian theologians like Tertullian (circa 160–225 CE) invoked Moloch in their condemnation of idolatry, positioning Christianity as a morally superior alternative. During the medieval era, various religious leaders used Moloch to critique the excesses of rival faiths, particularly in the context of the Crusades, where the practices of Muslims were often represented as Molochian sacrifices. Enlightenment figures such as Voltaire referenced Moloch to critique fanaticism, warning of how the thirst for power could lead to the sacrifice of reason and humanity. Empires and authoritarian regimes have deployed the Moloch myth to serve as a warning about humanity’s “darker impulses.”
This theme resonates in Allen Ginsberg’s Howl, published in 1956, where he invokes Moloch as a symbol of the oppressive, dehumanizing forces driving modern empires. In Ginsberg’s vision, Moloch embodies the machinery of modern power—capitalism, militarism, and industrialism—that sacrifices human potential for the sake of control, conformity, and violence, consuming the future of entire societies:
Moloch! Moloch! Nightmare of Moloch! Moloch the loveless!Mental Moloch! Moloch the heavy judger of men!
Moloch the incomprehensible prison! Moloch the crossbone soulless jailhouse and Congress of sorrows! Moloch whose buildings are judgement! Moloch the vast stone of war! Moloch the stunned governments!
Moloch whose mind is pure machinery! Moloch whose blood is running money! Moloch whose fingers are ten armies! Moloch whose breast is a cannibal dynamo! Moloch whose ear is a smoking tomb!
Moloch whose eyes are a thousand blind windows! Moloch whose skyscrapers stand in the long streets like endless Jehovahs! Moloch whose factories dream and croak in the fog! Moloch whose smokestacks and antennae crown the cities!
Moloch whose love is endless oil and stone! Moloch whose soul is electricity and banks! Moloch whose poverty is the specter of genius! Moloch whose fate is a cloud of sexless hydrogen! Moloch whose name is the Mind!
Moloch in whom I sit lonely! Moloch in whom I dream Angels! Crazy in Moloch! Cocksucker in Moloch! Lacklove and manless in Moloch!
Moloch who entered my soul early! Moloch in whom I am a consciousness without a body! Moloch who frightened me out of my natural ecstasy! Moloch whom I abandon! Wake up in Moloch! Light streaming out of the sky!22
Empires, both ancient and modern, have always pursued expansion, sacrificing other possible futures to conquest, war, and economic ambition. In today’s world, this drive is mirrored in a troubling trend, as empirically driven models of reality struggle to maintain authority over a rapidly shifting landscape. Humanity is now experiencing unprecedented ecological shifts, moving out of long-standing equilibriums and into “hysteresis loops”—unstable feedback cycles with unknown durations and outcomes, which disrupt our sense of direction. These changes unfold more slowly than rapid disruptions in the past have, such as meteor impacts or volcanic eruptions, but progress faster than gradual transformations like the Great Oxidation Event. As a result, the environmental conditions that sustain human life are being driven into uncharted extremes, intensifying both the urgency and challenges of scientific inquiry. Increasingly, science becomes an exercise in “dead reckoning,” where we gain knowledge of our current position but lack a clear sense of where these accelerating shifts are leading.
Geopolitics is grappling with the waning symbolic grip that empiricism supposedly has on reality, a shift reminiscent of earlier historical moments when changing scientific paradigms reconfigured international political orders and the logic of governance. As biological sciences reveal new layers of complexity and epistemological uncertainty, they highlight the need for governance frameworks that can account for interconnected and dynamic global crises, and that challenge traditional geopolitical models based on fixed hierarchies and causal clarity.23 Rather than engage with these intricacies, contemporary culture often mirrors past eras of scientific and political tension, sacrificing complexity for simplification—strategies historically employed to legitimize colonial domination or facilitate resource imperialism. This same destabilizing force is reshaping governance, creating new affordances for multipolar orders while simultaneously straining traditional models of alliances, roles, and hierarchies. Echoing historical multipolar traps described in systems of imperial resource exploitation and balance-of-power politics, causality models built on flawed or incomplete correlations undermine coordination efforts, creating destructive cycles of competition. In these conditions, Moloch traps—coordination failures that drive short-term optimization at the expense of long-term planning—emerge, mirroring industrial and resource-driven imperial strategies, where long-term governance goals are sacrificed for immediate gain.
I recently asked a leader of a research institution that works with global existential risk what most concerned them about the triple crisis of climate collapse, mass extinction, and rising underdetermination in the life and earth sciences. They answered that polarizing populism was the biggest threat to humanity in the twenty-first century:
If polarizing political figures and polarized populations turn against science in democratic systems—both because we can no longer promise absolute certainty and as a way to resist political change—then we are completely lost. I dread the desire to negotiate the most complex questions ever posed by a species to itself, through the binary choices crafted by contemporary populist narratives which exploit people’s fear for political advantages.
We must confront the twin forces of populism and existential uncertainty and pursue a path that recognizes the limits of knowledge without falling into either nihilism or naive solutionism. What do we stand to lose in a world that demands certainty yet faces growing unknowns? Are we doubling down on cultural games that preserve science’s authority over the knowable—even as these games fail to address the complexities of a rapidly evolving world? How might these historically familiar traps be sidestepped today, even as past steps have continually brought us back to them and have now brought us here? Underdetermination reminds us that the pursuit of knowledge has never been about eliminating uncertainty, but about navigating its profound depths.
Anaïs Nin, The Diary of Anaïs Nin, vol. 1, 1931–1934, ed. Gunther Stuhlmann (Harcourt Brace Jovanovich, 1966), entry for January1946.
Quentin Meillassoux, After Finitude: An Essay on the Necessity of Contingency, trans. Ray Brassier (Continuum, 2008), 128.
For an overview of the complexities and uncertainties in Arctic greening and an analysis of how vegetation changes are scale-dependent and tied to unpredictable environmental dynamics, see I. H. Myers-Smith et al., “Complexity Revealed in the Greening of the Arctic,” Nature Climate Change, no. 10 (2020).
A preliminary study of the timing of flowering season and pollination in the arctic can be found in Mark A. K. Gillespie and Elisabeth J. Cooper, “The Seasonal Dynamics of a High Arctic Plant-Visitor Network: Temporal Observations and Responses to Delayed Snow Melt,” Arctic Science 8, no. 3 (2022). An earlier analysis of potential ecosystem-wide disruptions and critical risks due to mismatches in Arctic plant and pollinator phenology can be found in N. M. Schmidt et al., “An Ecological Function in Crisis? The Temporal Overlap between Plant Flowering and Pollinator Function Shrinks as the Arctic Warms,” Ecography, no. 39 (2016).
For an introduction to the significance of DNA metabarcoding in biodiversity science, see K. Deiner et al., “Environmental DNA Metabarcoding: Transforming How We Survey Animal and Plant Communities,” Molecular Ecology 26, no. 21 (2017). For a more recent analysis of how pollen DNA metabarcoding is being used to understand the global restructuring of plant-pollinator networks and its potential for monitoring biodiversity under rapid environmental changes, see K. L. Bell et al., “Plants, Pollinators and Their Interactions under Global Ecological Change: The Role of Pollen DNA Metabarcoding,” Molecular Ecology 32, no. 23 (2023).
For an analysis of how Arctic warming disrupts plant-pollinator interactions, see N. M. Schmidt et al., “An Ecological Function in Crisis? The Temporal Overlap between Plant Flowering and Pollinator Function Shrinks as the Arctic Warms,” Ecography 39, no. 12 (2016). This article shows how temporal mismatches between flowering and pollinator activity threaten functional ecological stability.
This quote and all subsequent quotes emerged from writing this text (and two others) with the scientists mentioned.
Key insights into the challenges posed by “walls of complexity” in scientific inquiry can be found in, for example: Marta Bertolaso and Mattia Della Rocca, “On the Processes of Growth of the Living Being and the Relevance of Relational Categories to Understand It,” Rivista Di Filosofia Neo-Scolastica 109, no. 2 (2017): 262.
The evolving challenges of “peak complexity” in biology are comprehensively addressed in Philip Ball, How Life Works: A User’s Guide to the New Biology (University of Chicago Press, 2023). For an exploration of the theoretical dimensions driving the complexity crisis in cancer science, see Marta Bertolaso and Bernhard Strauss, “Redefining the Problem: The Theory Dimension of Cancer,” in Rethinking Cancer: A New Paradigm for the Postgenomics Era, ed. Bernhard Strauss et al. (MIT Press, 2021).
The first paper reporting on the quantity of microbial cells in the human gut was T. D. Luckey, “Introduction to Intestinal Microecology,” American Journal of Clinical Nutrition 25, no. 12 (1972).
Large metagenomic studies from European and American consortia include, for example, Junjie Qin et al., “A Human Gut Microbial Gene Catalogue Established by Metagenomic Sequencing,” Nature, no. 464 (2010); and The Human Microbiome Project Consortium, “Structure, Function and Diversity of the Healthy Human Microbiome,” Nature, no. 486 (2012).
The first paper describing the invention of the SHIME technology was K. Molly, M. Van de Woestyne, and W. Verstraete, “Development of a 5-step Multi-chamber Reactor as a Simulation of the Human Intestinal Microbial Ecosystem,” Applied Microbiology and Biotechnology 39, no. 2 (1993). A more recent analysis of in vitro simulations of the human gastrointestinal tract is Kristel De Paepe et al., “SHIME®: Simulator of the Human Intestinal Microbial Ecosystem,” Trends in Food Science & Technology, no. 116 (2021).
Arumugam’s reflection on the microbiome in evolutionary time finds ground in, for example, Howard Ochman and Allan C. Wilson, “Evolution in Bacteria: Evidence for a Universal Substitution Rate in Cellular Genomes,” Journal of Molecular Evolution 26, no. 1–2 (1987).
For an account of the development of single-cell sequencing technologies, see Axel A. Almet et al., “The Landscape of Cell-Cell Communication Through Single-Cell Transcriptomics,” Current Opinion in Systems Biology, no. 26 (2021).
See Farbod Shojaei and Napoleone Ferrara, “Role of the Microenvironment in Tumor Growth and in Refractoriness/Resistance to Anti-angiogenic Therapies,” Drug Resistance Updates 11, no. 6 (2008). For journalistic coverage, see “Cancer Drug Avastin Loses US Approval,” BBC News, November 18, 2011.
For a frequently cited review on the topic of the tumor microenvironment, see Kristian Pietras and Arne Ostman, “Introduction to Tumor-Stroma Interactions,” Experimental Cell Research 319, no. 11 (2013). A more recent overview is Karin E. de Visser and Johanna A. Joyce, “The Evolving Tumor Microenvironment: From Cancer Initiation to Metastatic Outgrowth,” Cancer Cell 41, no. 3 (2023).
Bonnie Shulman, “What If We Change Our Axioms? A Feminist Inquiry into the Foundations of Mathematics,” Configurations 4, no. 3 (Fall 1996).
In Indian philosophy, the Nyāya school systematically structures reasoning to ascertain truths through inference and sensory perception, promoting a nuanced understanding that deepens beyond simple binary distinctions of true or false. Jain philosophy’s Anekāntavāda emphasizes the many-sidedness of truth, and its syādvāda doctrine encourages recognizing partial truths and reconciling multiple viewpoints.
Daoist and Confucian traditions in China emphasize process-oriented views of reality. Daoism views objects as dynamic elements rooted in the constant flux and balance of the Dao, encouraging a perception of the world as interconnected processes. Confucian relational ontology prioritizes roles, relationships, and interdependence, centering understanding on dynamic interactions—such as those defined by familial duties or the practice of li (ritual propriety)—rather than fixed categories.
Shulman, “What If We Change Our Axioms?,” 442.
Shulman, “What If We Change Our Axioms?,” 451.
Allen Ginsberg, “Howl,” in Howl and Other Poems (City Lights Books, 1956), 17.
For a deeper analysis of the historical relationship between epistemic shifts in science and the international political order, see Bentley B. Allan, Scientific Cosmology and International Orders (Cambridge University Press, 2018).