Hijacking
In the twentieth century, the word “hijacking” came to typify the explanation of what viruses do to the cells they infect. How commonplace it is to now say: the virus hijacks the host cell’s machinery in order to replicate itself. You’ve heard a version of this narration, no doubt about it: viruses do not possess their own metabolisms and must rely on those of the beings they infect. They get energy and building materials from host cells to replicate their genetic sequences, repackaging those sequences into protein capsids and lipid envelopes for movement to the next host. In short, cells have metabolic machinery; viruses take it over and redirect its efforts toward making more viruses.
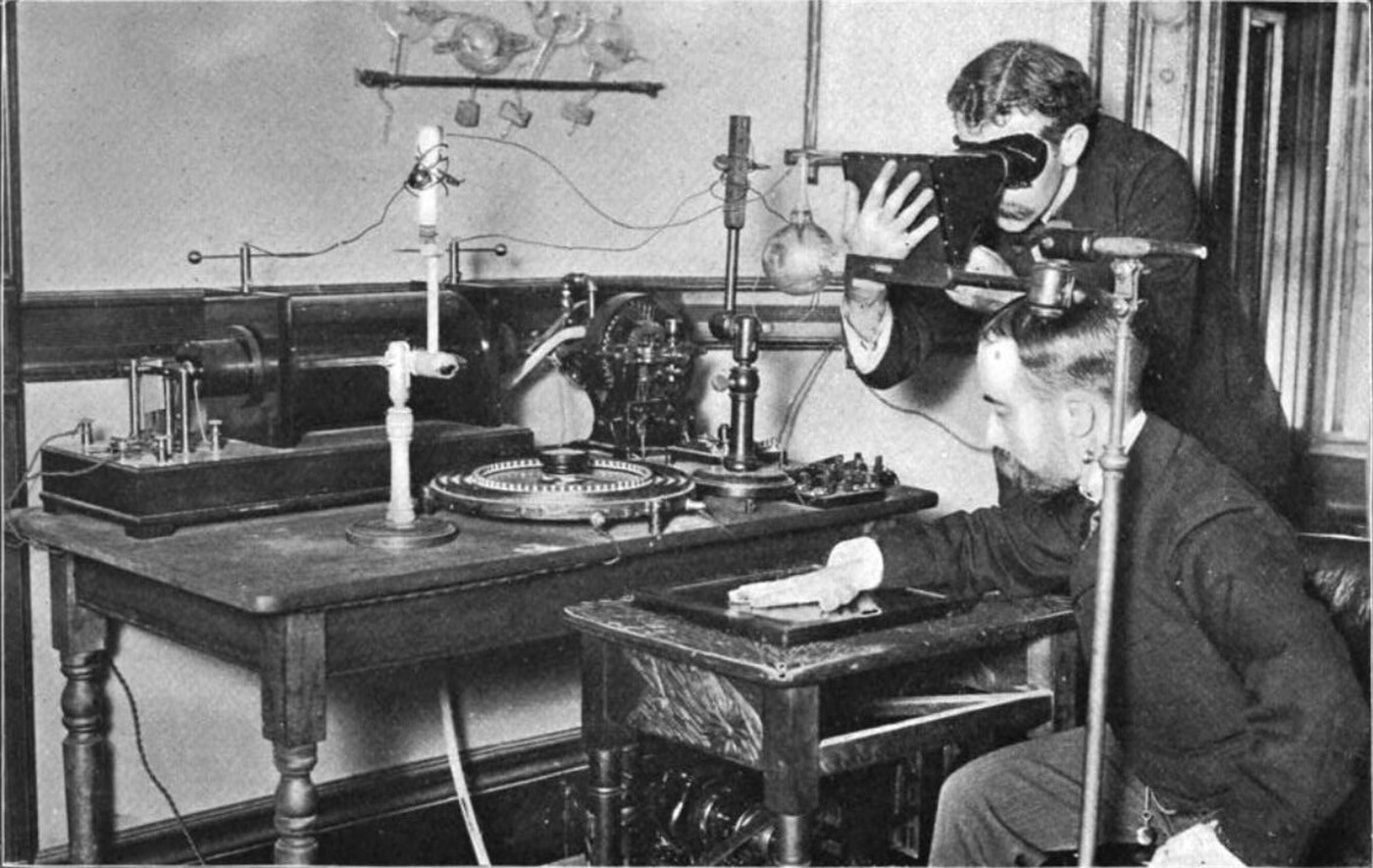
Taking an X-ray image with early Crookes tube apparatus, late 1800s. Licesne: public domain.
The word “hijack” is of roughly the same vintage as the concept that viruses are protected packages of genetic material. “Hijack” came into use from an unknown origin—potentially a combination of “highway” and “jacker” (as in carjacker) in American English in the early 1920s. Ships and trucks, and then later, planes, were hijacked. The use of x-ray diffraction in the 1930s to make the first images of a virus was interpreted as showing a protein shell encasing genetic cargo. The rhetorical construction of explanations for how viruses got around and how they were made coincided with the expansion of international networks and modes of shipping people, valuables, and factory-produced commodities around the globe—as well as their hijacking. Somewhat paradoxically, both viruses and the cells they infect were captured by this kind of vehicular representation, both the virus as a transport capsule protecting precious genetic cargo and the infected cell as a conveyance full of machinery that could be taken over. However, this overlap did not get in the way of the figuration of the virus as hijacker; perhaps there was enough science fiction imagery floating around (of little spaceships landing on big spaceships or planets and taking them over, for example) to provide a convenient way to mentally order the different parties.
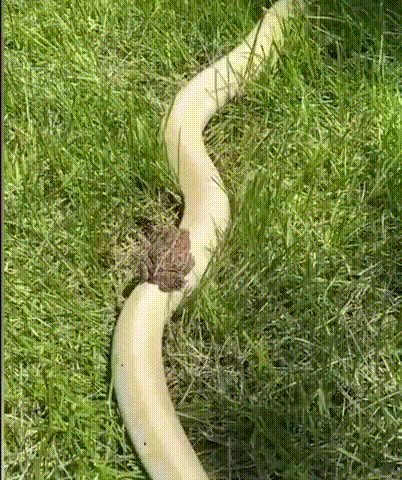
A frog rides snake like a train.
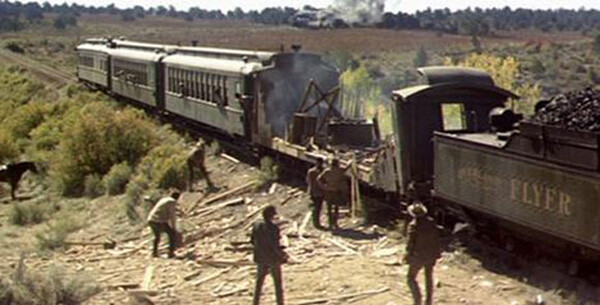
Train hijacking from Butch Cassidy and the Sundance Kid, 1969 (still).
A frog rides snake like a train.
However, viruses are not like spaceships, and cells are not just like twentieth-century semitrailer trucks, armored vehicles, or passenger jets whose resources can be plundered and whose operators can be coerced into unwanted journeys. As with many apparently innocuous explanatory tropes, this figure of the viral hijacker perhaps hides as much as it reveals. It transposes the idea of an individual or a group of individuals storming a vehicle and overwhelming its drivers and passengers by force; of a temporary and illegal deviation of a normal journey; of violence and coercion in the name of another cause. The hijacker is a terrorist, a thief, a stranger looking to take the wheel. It appears to transparently explain things. But is this a good description of a virus and the creatures it is capable of infecting? Perhaps we should try some other imagery on for size.
Maybe Viruses Are More Like Venomous Cone Snails Than Hijackers?
Anthropomorphism and personification of microbial entities in the explanation of virology is a understandable tendency; in this way, viral action is domesticated to the human scale. But maybe it’s time to practice some resolutely non-domesticating conid-amorphism, some conus-centrism, and see what happens if we forge a new avenue for thinking of viruses in terms of venomous cone snails. Conidae is the taxonomic name for the family of gastropods known more colloquially as cone snails, or predatory sea snails. “One of the most successful lineages of marine animals,” the hundreds of species of Conidae are characterized by their use of complex venoms to capture prey.1 Some of these snails prey on worms, some on other snails, and some on fish. In 2015, researchers reported that Conus geographus uses an insulin overdose to disorient and disable its fish prey, releasing the toxin into the water.
Insulin appears to be a component of the nirvana cabal, a toxin combination in these venoms that is released into the water to disorient schools of small fish, making them easier to engulf with the snail’s distended false mouth, which functions as a net. If an entire school of fish simultaneously experiences hypoglycemic shock, this should directly facilitate capture by the predatory snail.2
The released insulin does not affect the snail itself, because its venom insulin mimics fish insulin, not its own molluscan variety. Venomous snails that hunt worms in this fashion make a different insulin mimic, specific to worms.
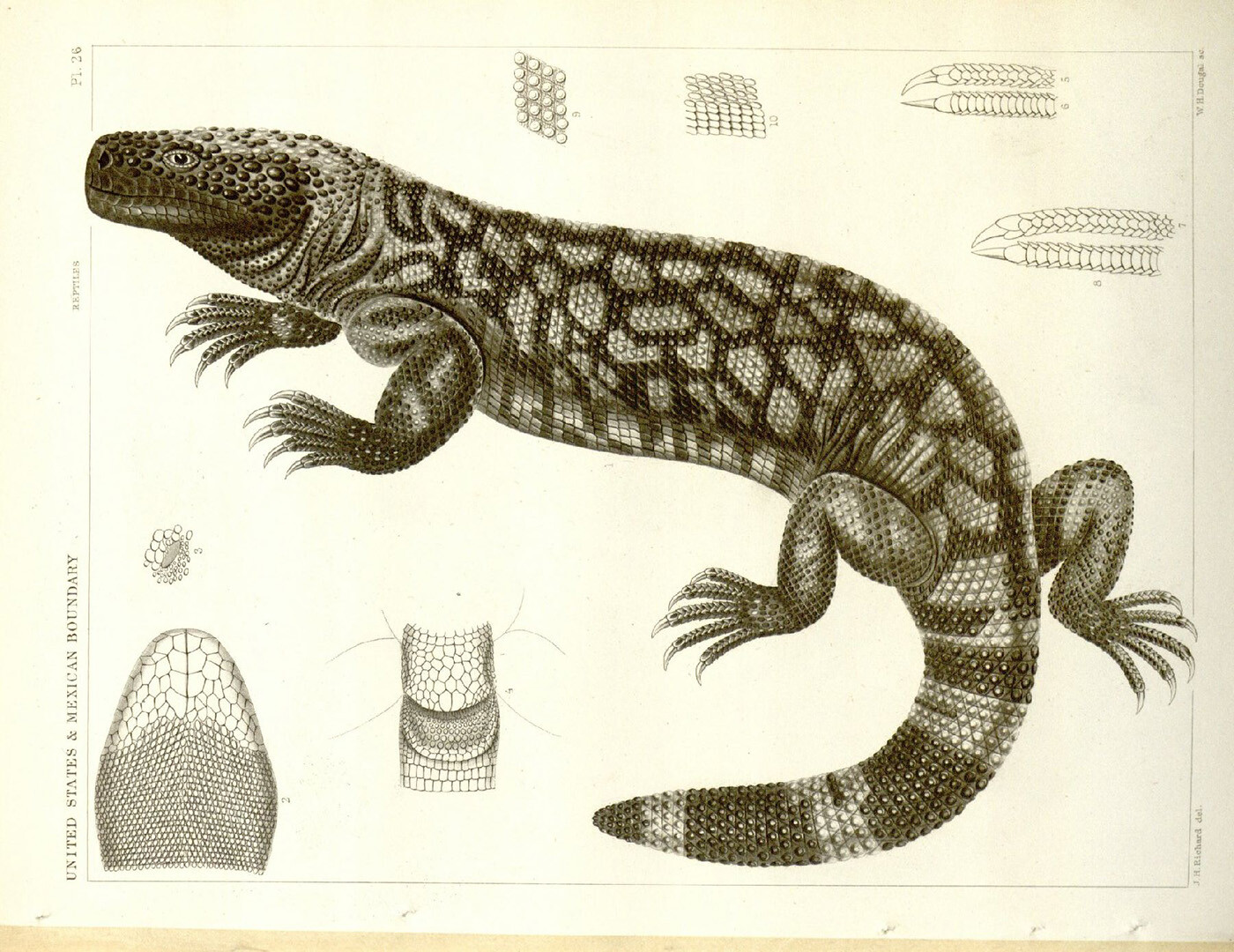
The first drawing of a Gila monster by Baird, S. F., 1857. License: CC BY-SA 4.0.
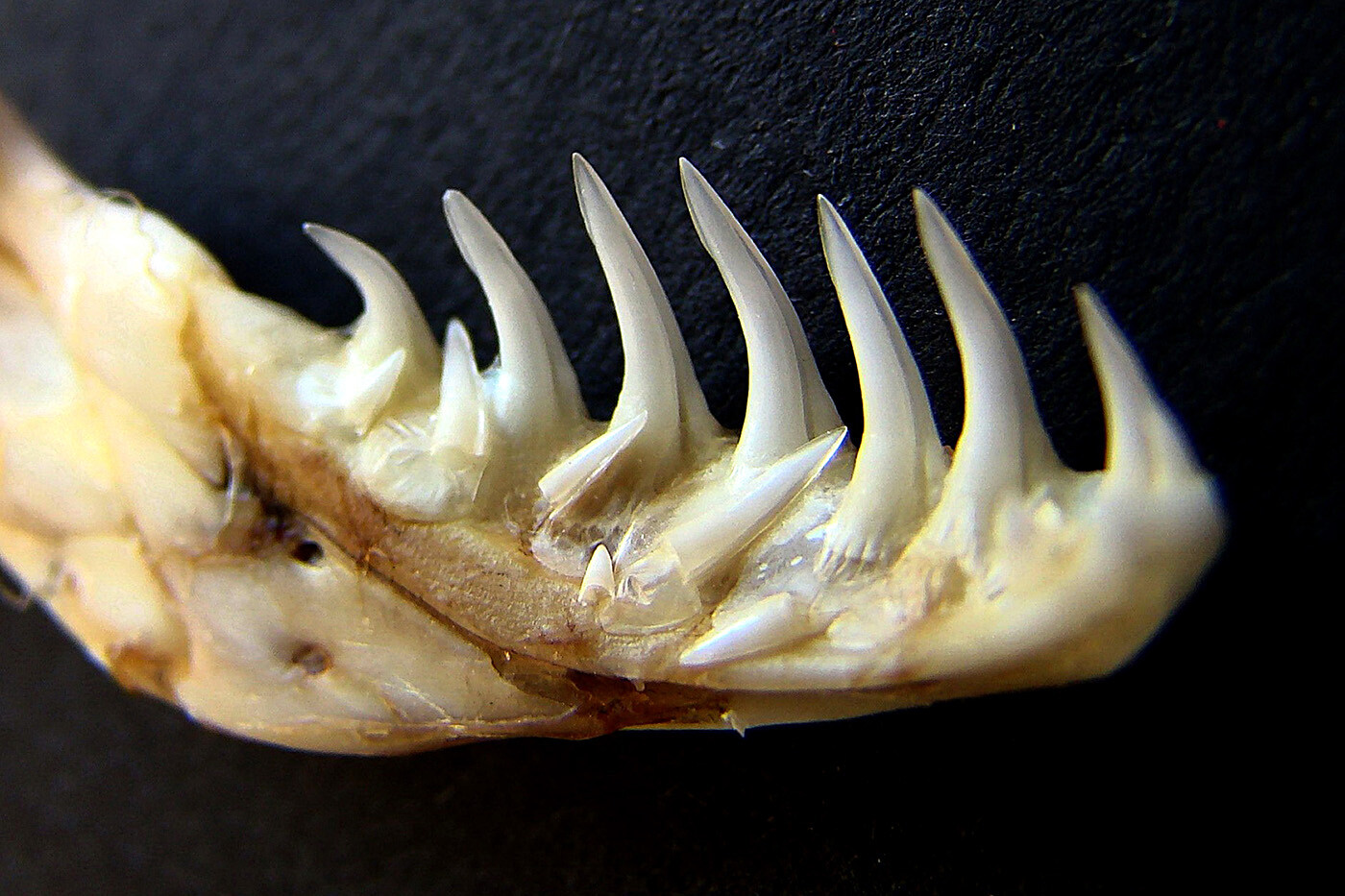
The grooves in a Gila monster’s teeth helps in spreading their venom into their prey. License: CC BY-SA 4.0.
The first drawing of a Gila monster by Baird, S. F., 1857. License: CC BY-SA 4.0.
This may seem like a weird aberration on the part of an obscure life-form, yet cone snails are only one kind of creature that roams the evolutionary space in which one being’s molecules evolve to participate in the physiology of another. There are enough examples of organisms employing these tactics that they are grouped together in scientific reviews of “weaponized hormones.”3 Elaborating molecular hormone mimics used by amphibians, mollusks, bacteria, viruses, and other (predatory) beings has recently become a handy drug-discovery strategy for pharmaceutical researchers. For example, the first diabetes therapy drug that works by mimicking the glucagon-like peptide hormone (GLP-1) was discovered by analyzing the venom of the Gila monster.4 GLP-1 is a hormone released by the gut after eating that stimulates insulin production and slows movement of food through the intestinal tract. The making of a drug that acts like GLP-1 was not a process of exactly copying human GLP-1. For that you wouldn’t need a Gila monster and thousands of years of coevolution between lizards and their prey. Rather, scientists learned alternative molecular strategies for binding to the hormone’s receptor from the Gila monster venom component.
Perhaps viruses are equally “interested” in glucose control in their own noncognitive way, in that their replication and continued existence is vested in their hosts’ metabolisms. As noted above, they do not possess the means for making the proteins encoded by the genes in their genomes, but rather depend on the cells they infect to do that translation and transcription. In order to make more of themselves and thus persist over time, viruses need rapid and high-intensity production of certain items such as lipids (fats) to make new viral particles, and a successful infection of a host cell is often followed by profound changes to the cell’s biosynthetic activity. Recently, an enterprising group of researchers noted that cells tend to use signaling molecules to cue themselves and each other to shift from states of quiescence to activity, or to ramp up or down various cellular products. They reasoned that viruses might get infected cells to shift into production mode by encoding these very signals. Looking in viral genomes for sequences akin to those that encode hormones, those researchers found what they were looking for, including a set of sequences that encode a molecule that looks (and acts) like insulin.5 Insulin is made in the beta cells of the human pancreas and is released into circulation after eating, where it signals to body cells to take up the glucose that is in the bloodstream after a meal. But it is also an ancient and evolutionarily conserved signaling system, with related versions of the molecule and its receptors found across the span of kingdoms, from the nematode worm with its forty or so insulin-like genes, to humans in which there is only one gene for insulin and a couple of insulin-like growth factors—and, of course, in cone snails and their devious insulins as well.
The family of viruses in which the researchers found these sequences encoding “virus insulin IGF-like peptides” (VILPs) is called the Iridoviridae, which are known to infect fish, but their DNA sequences have also been identified in the human gut.6 This tells us only that these viruses and their gene products may somehow interact with humans and their microbiome, not that they infect human cells. Indeed, it is not even clear whether the virus-encoded insulin-like peptide is helpful or harmful to its fish hosts, much less what it has to do with human health. After all, many bacterial symbionts of animals or plants make “eukaryote-like proteins” that mimic their hosts’ molecules in order to participate in their cellular processes, and this is one of the grounds of living together amicably with microbes rather than the interaction necessarily being one of disease.7 Regardless, the very fact that some viruses encode hormones, or “hormone-like” entities, is already quite surprising, enough so that one of the more difficult parts of the discovery was in even thinking to “search the viral genome for the presence of human-like hormones” in the first place.8 Why on earth would a virus carry genes that encode for hormones? They don’t even have their own bodies, for crying out loud. Well, that might actually be the answer. They don’t have their own bodies; they only have their hosts’ bodies. And bit by bit, through the endless nonillion-fold exploration of evolutionary space—the space that makes the difference between persisting long enough to be replicated versus falling apart into the wash of biological decay—it has turned out to be good practice to mimic your host’s cell cycle and metabolic hormones. Different viruses, different hosts, same strategy.
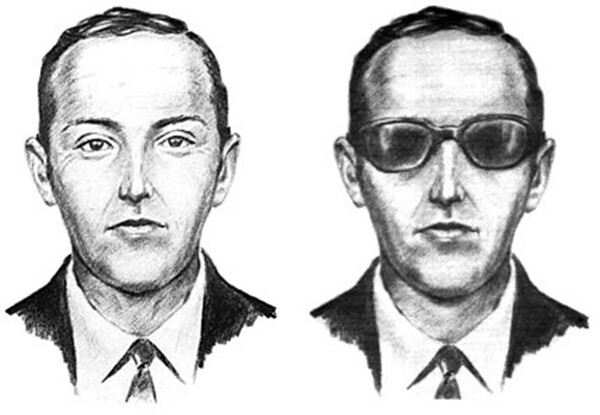
This FBI sketch shows the skyjacker D.B. Cooper, who bailed out of a Boeing 727 in 1971 and vanished with $200,000. He was never caught and the FBI announced in 2016 it was closing the case after 45 years. License: public domain.
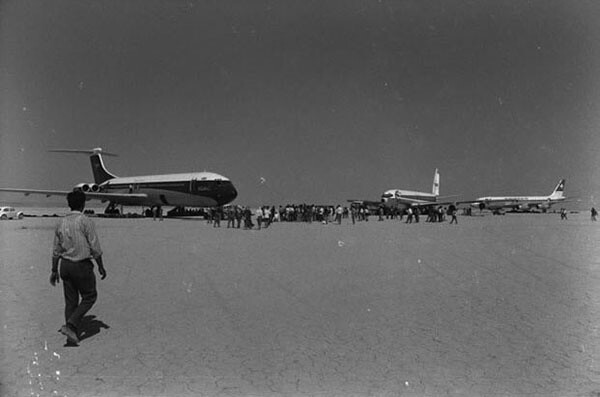
In September 1970, members of the Popular Front for the Liberation of Palestine (PFLP) hijacked four airliners bound for New York City and one for London. Three aircraft were forced to land at Dawson’s Field, a remote desert airstrip near Zarqa, Jordan, formerly Royal Air Force Station Zarqa, which then became PFLP’s “Revolutionary Airport.” License: public domain.
This FBI sketch shows the skyjacker D.B. Cooper, who bailed out of a Boeing 727 in 1971 and vanished with $200,000. He was never caught and the FBI announced in 2016 it was closing the case after 45 years. License: public domain.
On Having Proteins in Common: Kinship as Convergence and Divergence
Let us return to the idea that viruses are like cone snails, but instead of thinking metaphorically, we’ll proceed literally to ask how they are molecularly akin to one another, with their capacities to encode host-mimicking hormones. This is of course a scientific question, in that these relationships are only legible through the sequencing of genes, the bioinformatics by which databases of these gene sequences can be compared, or by the computational modeling and molecular biology that goes into understanding how a molecule can functionally or structurally mimic another even in the absence of any sequence similarity in the genes encoding them. At the same time, the dynamics of mimicry in living systems has long served as an enduringly provocative conceptual and theoretical resource—for biomimetics, for surrealism, for cultural anthropology, for ecology, and indeed for immunology itself, which has been debating the idea of “molecular mimicry” since it was coined in 1964. Perhaps the scientific story and its surprises can also be food for thought beyond the direct question of how viruses work, and can help inform a more expanded sense of how to live with, and among, this set of likenesses. Another way to put this is that instead of seeing viruses as being like (bad) human actors in a metaphorical sense (as in the hijacker analogy), perhaps there should be more energy and time invested in understanding how viruses, however unwelcome, are literally like humans—their protein kin. This is because we organisms are living together in a range of interlocking relations that range from the commensal to the predatory.
This relatedness is both genealogical and not. Viral hormone-like mimics are thought to arise from “divergent evolution,” a process in which a bit of the host genome is picked up and integrated into the viral genome during an infection event. This transferred gene does not remain the same; instead the two versions diverge over time, but there is nonetheless close sequence similarity, and the virally encoded protein is very similar to the host protein. On the other hand, mimicry might also arise through “convergent evolution,” where natural selection favors the makers of molecules that have structural or functional similarity to the host molecule. In the case of convergent evolution, the underlying genetic sequences are not similar at all, but the encoded molecule can have a close structure and function in terms of binding a receptor or some other physiologically consequential action.9 Thus, viruses take up, or converge, toward bits of their hosts.
Hosts also diverge to get away from their viruses if their own survival or fitness is compromised by infection. If organisms are targeted through the specifics of their hormones and receptors, a virus moving through a population acts as a selective pressure favoring changes to the target. This is called “adaptive evolution”; conservative estimates are that “30% of all adaptive amino acid changes in the conserved part of the human proteome” are driven by interactions with viruses.10 Meaning, viruses target proteins that are core to life functions, which tend to be conserved across evolution and therefore species; paradoxically, these are most likely to stay stable because of their importance, but also most likely to show adaptation once they become a landing pad enabling a virus to get into a cell. If viruses are effective because they mimic human hormones or hormone receptors, this will drive the evolution and duplication of hormones over time because it is advantageous for them to change enough to not be good viral targets. Thus, it has been suggested that some features of human hormonal genes in the placenta arise from their function as viral “decoys” in this tit-for-tat of copying and changing.11
It is for this reason that viruses could be thought of in terms of venomous cone snails rather than hijackers. Not all these mimicry techniques target metabolic processes; many viruses famously encode other proteins that allow them to evade immune detection by attempting to look like parts of the immune system rather than its targets. But there is a theme here. The virus takes on some aspects of the shape of the body that ensures its continuity. This gives rise to totally unlike beings containing patches of intimate and detailed sameness, molecules that bear the same precise contours to fit into a particular hormone receptor, yet perhaps bear no common genetic heritage. Viruses, like cone snails, evolve to be more like what sustains them. It is an uncomfortable form of relatedness, this predatory metabolic convergence, but it cannot be denied that it generates amazing patterns of likeness across biological kingdoms without everything having to be descended from the same line of direct genetic inheritance. Where does hormone end and hormone-like begin? If the mimic converges on the original, and places the original under evolutionary pressure to diverge, what is left is a seesawing mirrored relationship of competitive difference and similarity, not an original and a mimic. Even if something has evolved to get away from its mimic, it holds the imprint of that entity’s influence in its difference, like a shadow. In practical terms, looking into cone snails and viral genomes suggests new ways of making drugs, which are human-made mimics that seek to manipulate physiology by augmenting or suppressing the action of the molecule that has been mimicked. In philosophical terms on the other hand, that cone snail or amphibian venom gets enrolled in the diabetes epidemic by becoming a blood sugar–controlling drug indicates that anthropomorphic concepts such as hijacking may possibly be the least illuminating explanatory tactic for understanding what viruses do, and are, in relation to their bodies. (Yes, their bodies. That means us).
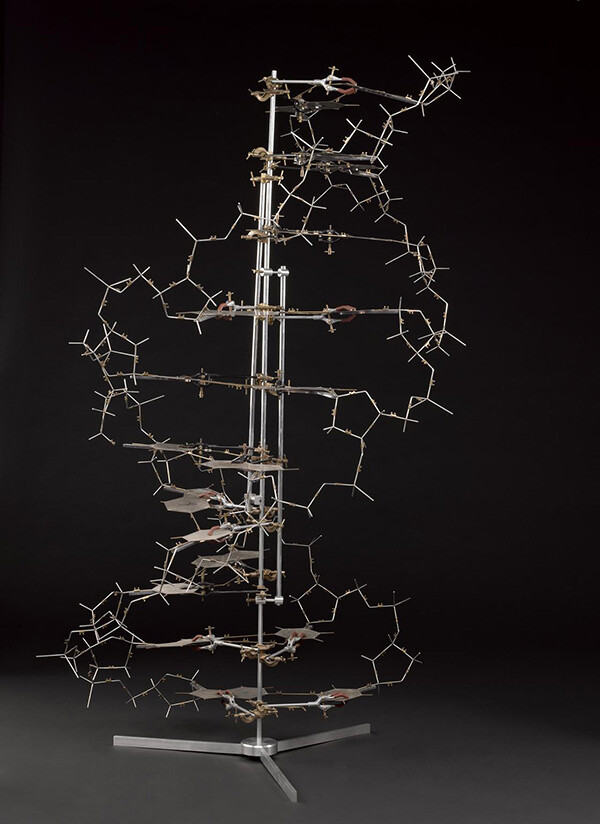
Reconstruction of the double helix model of DNA built by Francis Crick and James Watson in 1953. Photo: The Board of Trustees of the Science Museum.
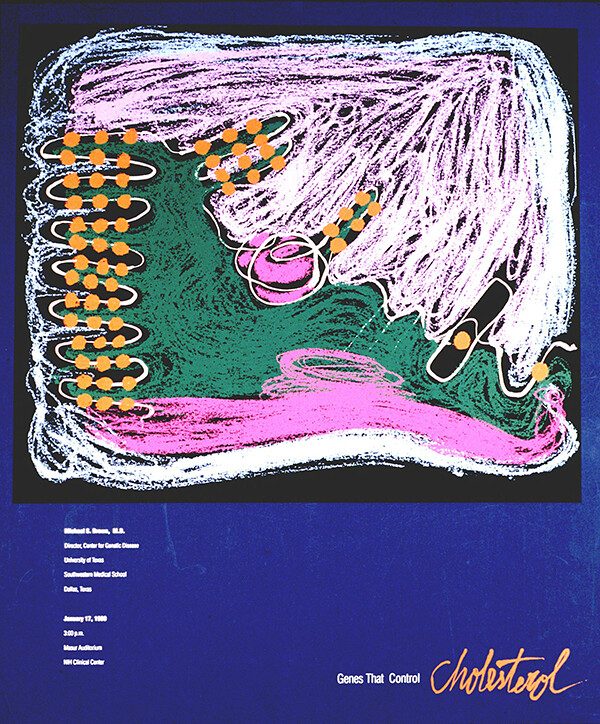
Genes that control cholesterol. Source: The National Library of Medicine. License: public domain.
Reconstruction of the double helix model of DNA built by Francis Crick and James Watson in 1953. Photo: The Board of Trustees of the Science Museum.
The Metabolic Body of Covid-19
As I noted above, the idea of viruses as foreign agents that forcibly take over the “cellular machinery” in a kind of illicit raiding operation is a distinctively mid-twentieth-century construct, dating to the formulation of what historians of science call the modern virus concept: a DNA or RNA core contained in a protective package transmittable across time and space between and within susceptible hosts. This virus concept is tightly bound up with that of the gene and its material basis as it developed over the twentieth century. Just when agreement was consolidating around DNA as the material basis of heredity, viruses took center stage as “defining representatives of ‘infective heredity’”—heredity that could be passed between cells.12
Within the context of an increasing uptake of the distinctions made by cybernetics between information and energy in the 1950s and ’60s, viruses seemed a prime example of how the information structure of a system dominated the dispensation and flow of energy. In the second edition of Cybernetics published in 1961, Norbert Weiner asks about the philosophical difference between a cybernetic control system and a virus that “guides into its own form other molecules of the same virus out of the tissues and juices of its host.”13 Juices! This seems more like a butcher talking about meat than a scientist talking about biology. Could we see any more disdain for the specificity of the raw material to be given form? Given this context, the genetic study of viruses dominated virology, and the dynamics of the recognition, memory, and specific targeting of viruses and virus-infected cells by the immune system likewise dominated the study of immune mechanisms in infectious disease.
Within this larger field of virus studies, during this period a very small number of researchers became interested in the question of what happened to the host’s metabolism when infected by a virus. Part of this interest was practical: in the effort to set of host-virus model systems in tissue culture and embryonated eggs, it was important to maintain the life of the host cells adequately for the production of virus. If you didn’t know how to manage it, you just ended up with dead tissue and no virus to work on. But some of the interest in “virus metabolism”—as the studies came to be called—came from curiosity about the tendency of viruses to sediment together with mitochondria when infected cells were centrifuged, and parallels between the virus-infected cell and the cancer cell both showing disordered growth accompanied by profound metabolic change. A minor trickle of publications looking at changes in cellular metabolism on infection in tissue culture and egg systems in the 1950s and ’60s established the view that most viruses induced a shift to glycolysis in infected cells as the main route for the production of ATP (Adenosine triphosphate), increasing uptake of glucose. Yet these remained for the most part footnotes to the increasing focus in virology on the virus as an agent of genetic control that changed a downstream metabolic program, enhanced even further by the intentional use of retroviruses as mutational agents in cancer research and as vectors for genetic engineering. Viral metabolism, or the human-viral metabolic relation, languished almost entirely until picking up again around 2010.
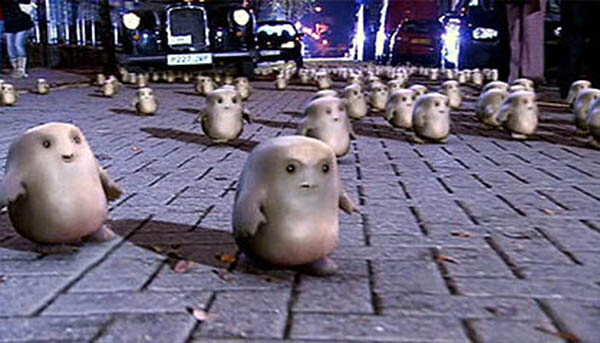
The Adipose, the antagonists of “Partners in Crime,” an episode of Doctor Who (still). They are named after a fat tissue in the human body.
It always feels a bit foolish, standing around critiquing the rhetoric of scientific explanation while seemingly more consequential matters are afoot. Yet one cannot survey the outcomes of two years of SARS-Cov-2 moving through human populations without being shaken by the profundity with which metabolic health stratified by race and class was a determinant of who died or suffered the severest outcomes, and how surprising this seems to have been to the research community. From the very outset of the pandemic in China, and echoed and confirmed in populations in Europe, the United Kingdom, India, Brazil, and North America, the disproportionate impact of the virus in individuals with so-called “underlying” metabolic conditions such as diabetes and hypertension has been clear. While the counting techniques of cases and conditions vary, and disagreements are rampant about what factors are most relevant to severe symptoms and fatality, preexisting poor blood sugar control, adiposity, and fatty liver disease all made the course of infection more likely to be fatal, and recovery harder across the board. A cruel irony is that not only is metabolic dysfunction a risk for Covid, but it is also a risk from it. Infection with SARS-Cov-2 can cause the novel onset of diabetes in patients who previously were not diabetic. Profound impacts on the usual homeostatic ups and down of making and using cellular energy in mitochondria are one of the factors contributing to the exercise-induced fatigue seen in patients suffering long Covid symptoms. And these factors in turn can make these individuals suffer higher risks of recurring breakthrough infections, even after vaccination. In short, immune and metabolic function are intimately entwined, just as infection and metabolism are intimately entwined.14
There is still much confusion in how to understand the Covid-19 pandemic, in part because viral theory doesn’t have much cushion to fall back on when it comes to these viral-immune-metabolic relations. Public health services have spent decades trying to convince people that metabolic disorders are the consequences of individual behavioral choices (smoking, poor diet) rather than the result of poor environmental conditions, economic and geographic displacement, and so on; viral infections are in another department altogether in the stark categories between chronic and infectious processes. Researchers have performed remarkably complicated micro-analyses of molecules and meta-analyses of thousands of research papers linking Covid-19 dynamics to metabolic disorder. Yet when interviewed about the implications of their findings, these researchers are still unable to say anything more sophisticated than voicing the sentiment that people need to eat better and exercise more, or that governments need to do a better job of regulating people’s sugar intake through taxation. Commentators still remark on the “collision” of two pandemics: a “syndemic” of acute illness in a population suffering chronic illness, which implies that these are two separate and unrelated phenomena that intersect.15
Let me be very clear: the finding of viral hormones in an apparently remote group of fish viruses discussed above has no direct bearing on these questions. There is no indication that coronaviruses make metabolic hormone mimics, and it is still unclear why, exactly, obesity and diabetes are risk factors for severe disease course. Maybe it’s just sheer coincidence and tragically bad luck that this particular virus, SARS-Cov-2, is especially harsh in its effects given the relatively recent world-historical shift in blood sugar, adiposity, and chronic inflammation as many populations age. Yet at the same time it is evident that understanding viruses as energetic and metabolic relations—both in the sense of how two or more concepts or objects are related, and in the sense of being an evolutionary relative connected by divergent or convergent change over time—has taken a backseat in research for as many decades as the virus has been a microscopically and genetically identified entity. That is why researchers and scientists, as well as the general public, need to think more about venomous cone snails and less about hijackers, more about the uncomfortable embrace of likeness arising from mimicry and evasion and less about command-and-control systems: because we have all been left collectively unprepared to understand and act on SARS-Cov-2 and its particular metabolic body, much less the ramifying interconnections this sets up between viral dynamics and the social organization of metabolic health.
Sophie Heiden Laugesen, Danny Hung-Chieh Chou, and Helena Safavi-Hemami, “Unconventional Insulins from Predators and Pathogens,” Nature Chemical Biology 18, no. 7 (July 2022): 689.
Helena Safavi-Hemami et al., “Specialized Insulin Is Used for Chemical Warfare by Fish-Hunting Cone Snails,” PNAS 112, no. 6 (2015): 1743.
Laugesen, Chou, and Safavi-Hemami, “Unconventional Insulins.”
Brian L. Furman, “The Development of Byetta (Exenatide) from the Venom of the Gila Monster as an Anti-Diabetic Agent,” Toxicon 59, no. 4 (2012): 464–71.
Emrah Altindis et al., “Viral Insulin-like Peptides Activate Human Insulin and IGF-1 Receptor Signaling: A Paradigm Shift for Host–Microbe Interactions,” Proceedings of the National Academy of Sciences 115, no. 10 (2018): 2461–66.
Khyati Girdhar et al., “Viruses and Metabolism: The Effects of Viral Infections and Viral Insulins on Host Metabolism,” Annual Review of Virology 8, no. 1 (2021): 373–91.
Louis J. Cohen et al., “Commensal Bacteria Make GPCR Ligands That Mimic Human Signalling Molecules,” Nature 549, no. 7670 (September 2017): 48–53.
Altindis et al., “Viral Insulin-like Peptides,” 2461.
Qian Huang, C Ronald Kahn, and Emrah Altindis, “Viral Hormones: Expanding Dimensions in Endocrinology.” Endocrinology 160, no. 9 (2019): 2165–79.
David Enard et al., “Viruses Are a Dominant Driver of Protein Adaptation in Mammals,” ELife, no. 5, ed. Gilean McVean (2016): 13.
Michael Wallis, “Do Some Viruses Use Growth Hormone, Prolactin and Their Receptors to Facilitate Entry into Cells?” BioEssays 43, no. 4 (2021).
Angela N. H. Creager, The Life of a Virus: Tobacco Mosaic Virus as an Experimental Model, 1930–1965 (University of Chicago Press, 2002), 244.
Norbert Wiener, Cybernetics Or Control and Communication in the Animal and the Machine (MIT Press, 1965), 180.
Norbert Stefan, “Metabolic Disorders, COVID-19 and Vaccine-Breakthrough Infections,” Nature Reviews Endocrinology 18, no. 2 (February 2022): 75–76.
Norbert Stefan, Andreas L. Birkenfeld, and Matthias B. Schulze, “Global Pandemics Interconnected — Obesity, Impaired Metabolic Health and COVID-19,” Nature Reviews Endocrinology 17, no. 3 (March 2021): 135–49; Merrill Singer et al., “Syndemics and the Biosocial Conception of Health,” The Lancet 389, no. 10072 (2017): 941–50.